The phosphorus atom
is one of living matter's basic building blocks. It is present
in every living creature and in every reef aquarium's water.
Unfortunately, it is often present in excess in reef aquaria,
and that excess has the potential to cause at least two substantial
problems for reefkeepers. The first is that phosphate is often
a limiting nutrient for algae growth, so when elevated it
can permit excessive growth of undesirable algae. The second
is that it can directly
inhibit calcification by corals and coralline algae. Because
most reefkeepers don't want either of these things to happen,
they strive to keep phosphorus levels under control. Fortunately,
there are several effective ways to keep phosphorus concentrations
at acceptable levels. What these methods are, and what are
appropriate goals for reef aquarium phosphate concentrations
will be addressed in this article.
Phosphorus exists in two primary forms
in seawater: as inorganic phosphates, especially orthophosphate,
and as organophosphate forms. Orthophosphate is readily taken
up by algae and is active in inhibiting calcification. The
organic forms may or may not be available to organisms such
as algae. Aquarists can readily test for inorganic orthophosphate
using a standard aquarium phosphate test kit, but testing
for organic phosphorus compounds is considerably more tedious.
Moreover, if there is an algae problem, then the algae may
be consuming the orthophosphate as fast as it enters the water,
thereby masking the issue. Consequently, many reef aquarists
may not recognize that they have a phosphate problem, only
that they have an algae problem.
This article describes some of the issues surrounding phosphorus
in reef aquaria, including the forms that it takes, its origins,
ways to test for it and, most importantly, ways to export
it.
The sections of this article are:
Phosphate in Seawater
The "simplest" form of phosphorus
in seawater is inorganic orthophosphate (sometimes called
Pi by biologists). It consists of a central phosphorus atom
surrounded by four oxygen atoms in a tetrahedron (Figures
1 and 2). Three of these oxygen atoms can either have an attached
hydrogen atom or carry a negative charge (Figure 2). The ratio
of these different forms depends on the pH in seawater. At
pH 8.1 seawater contains 0.5% H2PO4-,
79% HPO4-- and 20% PO4---.
At higher pH the equilibrium shifts toward more PO4---
and less HPO4--. For a variety of reasons,
especially including the ion pairing and consequent stabilization
of PO4--- by calcium and magnesium,
there is far more PO4--- in seawater
than in freshwater at the same pH. This shift in phosphate
species distribution with pH may seem esoteric, but it actually
has important implications for such things as the binding
of phosphate to calcium carbonate rock and sand, because the
different forms bind to different extents.
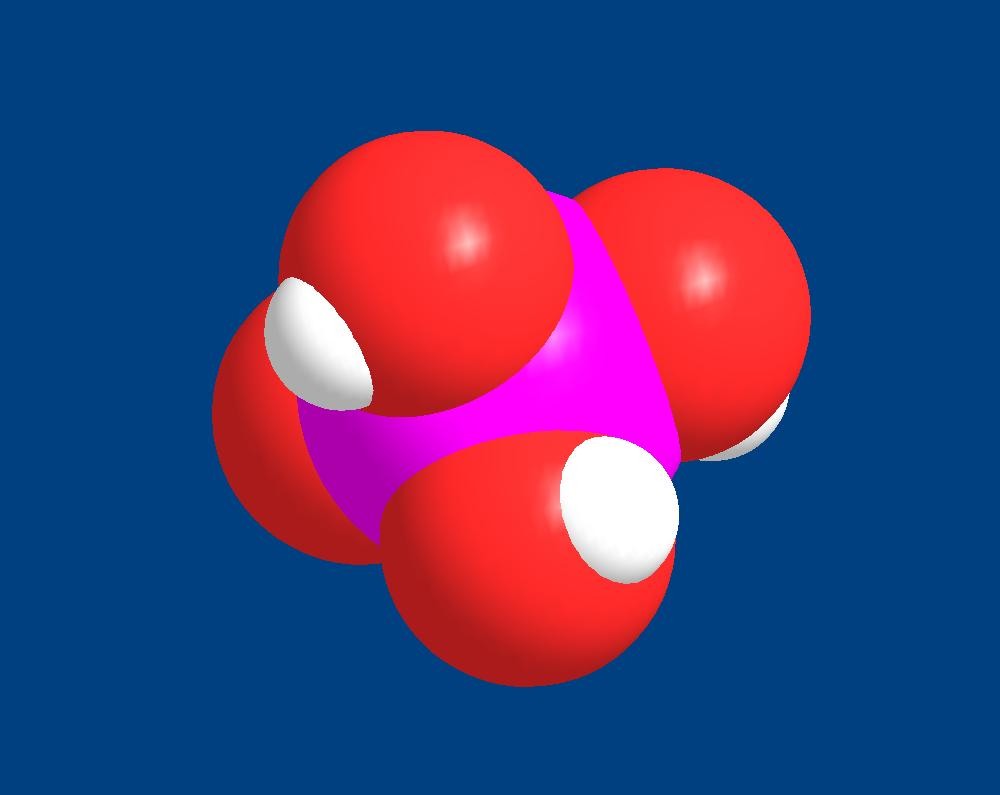 |
Figure 1. The three dimensional structure of
inorganic orthophosphate, shown in a fully protonated
form. It is comprised of a central phosphorus atom (purple)
and four oxygen atoms (red) arranged in a tetrahedron.
Three of the oxygen atoms are shown with an attached
proton (white).
|
Figure 2. The structure of inorganic orthophosphate,
with a central phosphorus atom (purple) and four oxygen
atoms arranged in a tetrahedron. Three of the oxygen
atoms can either have an attached proton (green) or
be present with a negative charge on the oxygen atom
(red). The amount present in each form in seawater varies
with pH as indicated.
|
The orthophosphate concentration in the ocean varies greatly
from place to place and also with depth and time of day. Surface
waters are greatly depleted in phosphate relative to deeper
waters, due to biological activities that sequester phosphate
in organisms. Typical ocean surface phosphate concentrations
are very low by reefkeeping standards, sometimes as low as
0.005 ppm.1
At concentrations below about 0.03 ppm, the growth rate of
many phytoplankton species depends on the phosphate concentration
(assuming that something else, such as nitrogen or iron, is
not limiting their growth). Above this level, many organisms'
growth rate is independent of phosphate concentration.1
So, to deter algal growth by controlling phosphate, aquarists
need to keep the phosphate levels quite low.
Other Forms of Inorganic Phosphate
Phosphorus also can take other inorganic
forms, such as the polyphosphates, which are rings and chains
of phosphate ions strung together by P-O-P bonds. While these
are usually insignificant in natural seawater, they can be
present in various solutions that are added to reef aquaria.
There are many of these compounds, but most will likely break
down into orthophosphate when added to a reef aquarium. Polyphosphates
are used industrially to bind metals, such as in some laundry
detergents. In that application they form soluble complexes
with calcium and magnesium, softening the water and enhancing
cleaning action. The amount of phosphate entering natural
waterways from laundry detergents, however, is high enough
that algae blooms sometimes result, and the practice of using
phosphate in detergent is now illegal in many places.
Organic Phosphates
In seawater, organic
phosphorus compounds are far more varied and complex than
inorganic phosphates. Many common biochemicals contain phosphorus
and every living cell contains a wide variety of them. Molecules
such as DNA, ATP, phospholipids (lecithin) and many proteins
contain phosphate groups. In these molecules, the basic phosphate
structure is covalently
attached to the remainder of the organic molecule through
one or more phosphate ester bonds to a carbon atom.
These bonds are stable for some period of time in water,
but eventually break down to release inorganic orthophosphate
from the molecule's organic part, a process that can be sped
up through the action of enzymes in a reef aquarium. Many
of these organic phosphate compounds will be readily removed
from an aquarium by skimming.
Export of organic phosphates is likely the major way that
skimming can reduce inorganic orthophosphate levels in an
aquarium. Orthophosphate ions are not significantly removed
via skimming (because they do not adsorb onto an air/water
interface), but organic phosphates can be removed before they
are converted into inorganic orthophosphate.
An important point about organic phosphates is that they
are not readily bound by inorganic phosphate-binding materials
used in the aquarium hobby. Consequently, while these products
may do a fine job of reducing inorganic orthophosphate, they
may not substantially reduce organic phosphates.
A final point is that organic phosphates are not detected
by most test kits designed for hobbyists. Those that do detect
organic phosphates (e.g., Hach PO-24) break the phosphate
off the organic compound, thereby converting it into inorganic
orthophosphate prior to testing. These kits are tedious to
use and expensive, however, so they're not for every hobbyist.
Indeed, I've never used one.
Organics in seawater are often measured in terms of their
nitrogen content, such as dissolved organic nitrogen (DON)
and particulate organic nitrogen (PON). The same is true for
phosphorus, using the terms dissolved organic phosphorus (DOP)
and particulate organic phosphorus (POP). Table 1 shows the
relative concentrations of C, N and P in typical dissolved
organic material found in seawater.1 In dissolved
organic material, nitrogen is about tenfold less prevalent
than carbon, and phosphorus is several hundredfold lower in
concentration than carbon.
Table
2. Elemental Composition of
Dissolved Organic Material.
|
Component:
|
Concentration:
|
DOC
|
60-90
mM
|
DON
|
3.5-7.5
mM
|
DOP
|
0.1-0.4
mM
|
DOC:DON
ratio |
9-18
|
DOC:DOP
ratio |
180-570
|
|
Phosphate Sources in Reef Aquaria
Organic phosphorus compounds, as well
as orthophosphate, are so prevalent in biological systems
that any natural food necessarily contains significant concentrations
of phosphorus. Not only can organic material be taken up directly
to provide carbon, nitrogen and phosphorus, it can be broken
down by organisms and released as inorganic nutrients, such
as orthophosphate, ammonia, nitrite and nitrate. The metabolic
breakdown scheme for typical organic materials in phytoplankton1
is shown below:
(CH2O)106(NH3)16(H3PO4)
+ 138 O2
à
106 CO2
+ 122 H2O
+ 19 H+ + PO4---
+ 16 NO3-
organic + oxygen à
carbon dioxide + water + hydrogen ion + phosphate + nitrate
Flake fish food is typically about 1% phosphorus (3% phosphate
equivalent) by weight (and many products have such phosphorus
data on their labels). Consequently, if five grams of flake
food is added to a 100-gallon aquarium, there is the potential
for the inorganic orthophosphate level to be raised by 0.4
ppm in that SINGLE FEEDING! That fact can be a significant
issue for reefkeepers: what do we do with all of that phosphorus?
If the food is completely converted into tissue mass, then
there will be no excess phosphate. But much of the food that
any heterotrophic organism consumes goes to provide energy,
leaving a residue of CO2
(carbon dioxide), phosphate and a variety of nitrogen-containing
compounds (ammonia, nitrite, nitrate, etc.) as shown above.
A fish, whether it is an adult or a growing juvenile, consequently
excretes much of the phosphorus that it takes in with its
food as phosphate in its waste. Of course, overfeeding will
result in more phosphate delivery than will reduced feeding
levels.
Unfortunately, many types of seafood available at the grocery
store have various inorganic phosphate salts intentionally
added to them as preservatives. These foods include canned
and frozen seafood, as evidenced by their label, and even
some fresh seafood. In these cases, rinsing the food before
using it may help to reduce the phosphate load it adds to
the aquarium.
Finally, tap
water can also be a significant source of phosphate. The
tap water that the Massachusetts Water Resources Authority
supplies to me is acceptably low in phosphate, or at least
it was the last time I measured it. In other water supplies,
however, phosphate levels can be too high for reefkeeping.
In 2005 New
York City officials reported that water samples showed
phosphate levels as high as 3 ppm. I'd recommend phosphate
testing to anyone with an algae problem who uses tap water,
in order to ascertain whether phosphate in the water is an
issue.
On the other side of this issue are those reef aquaria without
fish. Because phosphorus is required for growing tissue, it
is mandatory that some phosphorus source be available to corals
and invertebrates growing in a reef aquarium. Finding a source
is trivial if fish are in the aquarium, but in aquaria without
fish, reefkeepers must somehow add phosphorus. The solution
to this problem is easy: either add fish food, even though
there are no fish, or add a source of phosphorus such as a
plant fertilizer (and if the fertilizer does not contain nitrogen,
a source of that may be needed as well).
Calcification Inhibition by Phosphate
One important issue relating to elevated
phosphate in reef aquaria has to do with the inhibition
of calcification by phosphate and phosphate-containing
organics. Phosphate is known to inhibit the precipitation
of calcium carbonate from seawater.2-4 The presence
of phosphate in the water also decreases calcification in
corals, such as Pocillopora damicornis5
and entire patch reefs.6 This inhibition is likely
related to the presence of phosphate in the extracytoplasmic
calcifying fluid (ECF), where calcification takes place in
corals7, and on the growing crystal's surface.
Exactly how the phosphate gets into the ECF isn't well understood.
Figure 3. The chemical structure of the organophosphate
"etidronate," shown in a fully protonated
form.
|
|
This inhibition of calcification takes place at concentrations
frequently attained in reef aquaria, and may begin at levels
below those detectable by hobby test kits. For example, one
research group found that long-term enrichment of phosphate
(0.19 ppm; maintained for three hours per day) on a natural
patch reef on the Great Barrier Reef inhibited overall coral
calcification by 43%.6 A second team found effects
in several Acropora species at similar concentrations.8
Organic phosphate and phosphonate inhibitors of calcification
have also been studied and probably work by a similar mechanism.
Etidronate,
a bisphosphonate that is used to treat osteoporosis (Figure
3), caused a 36% inhibition of calcification in Stylophora
pistillata at 2 ppm, and stopped it completely (99%) at
100 ppm, while photosynthesis was not impacted at these, and
higher, concentrations (indicating it is not a general toxin).9
How to Export Phosphate
Now that we know where phosphate comes
from, and what impact it has, we can proceed to ask where
it goes and how to maximize those export processes. Certainly,
some phosphorus goes into the bodies of growing organisms,
including bacteria, algae, corals and fish. Some of these
organisms stay permanently in the aquarium, and others may
be removed by algae harvesting, skimming of small organisms
and even pruning of corals. These and other mechanisms are
discussed in subsequent sections of this article.
Phosphate Reduction via Calcium
Phosphate Precipitation
One mechanism for phosphate reduction
in reef aquaria may simply be the precipitation of calcium
phosphate, Ca3(PO4)2. The
water in many reef aquaria is supersaturated with respect
to this material, as its equilibrium saturation concentration
in normal seawater is only 0.002 ppm phosphate. As with CaCO3,
the precipitation of Ca3(PO4)2
in seawater may be limited more by kinetic factors than by
equilibrium factors, so it is impossible to say how much will
precipitate under reef aquarium conditions (without, of course,
somehow determining it experimentally). This precipitation
may be especially likely where calcium and high pH additives
(such as limewater) enter the aquarium water. The locally
high pH converts much of the HPO4--
to PO4---. Combined with the locally
high calcium level (also from the limewater), the locally
high PO4--- level may push the supersaturation
of Ca3(PO4)2 to unstable
levels, causing precipitation. If these calcium phosphate
crystals are formed in the water column (e.g., if they form
at the local area where limewater hits the aquarium water),
then they may become coated with organics and be skimmed out
of the aquarium.
Many reefkeepers accept the concept that adding limewater
reduces phosphate levels. This may be true, but the mechanism
remains to be demonstrated. Craig Bingman has done a variety
of experiments related to this hypothesis, and has published
them in the old Aquarium Frontiers magazine. While
many aquarists may not care what the mechanism is, knowing
how it occurs will help us understand the limits of this method,
and how to best employ it.
One possible mechanism could be through calcium phosphate
precipitation, as outlined above. A second mechanism for potential
phosphate reduction when using high pH additives is the binding
of phosphate to calcium carbonate surfaces. The absorption
of phosphate from seawater onto aragonite is pH dependent,
with the binding
maximized at around pH 8.4 and with less binding occurring
at lower and higher pH values. Habib Sekha (owner of Salifert)
has pointed out that limewater additions may lead to substantial
precipitation of calcium carbonate in reef aquaria. This idea
makes perfect sense. After all, it is certainly not the case
that large numbers of reef aquaria exactly balance calcification
needs by replacing all evaporated water with saturated limewater.
And yet, many aquarists find that calcium and alkalinity levels
are stable over long time periods with just that scenario.
One way this can be true is if the excess calcium and alkalinity,
which such additions typically add to the aquarium, are subsequently
removed by precipitation of calcium carbonate (such as on
heaters, pumps, sand, live rock, etc.). It is this ongoing
precipitation of calcium carbonate, then, that may reduce
the phosphate levels; phosphate binds to these growing surfaces
and becomes part of the solid precipitate.
If the calcium carbonate crystal is static (not growing),
then this process is reversible, and the aragonite can act
as a reservoir for phosphate. This reservoir can inhibit the
complete removal of excess phosphate from a reef aquarium
that has experienced very high phosphate levels, and may permit
algae to continue to thrive despite all external phosphate
sources having been cut off. In such extreme cases, removal
of the substrate may even be required.
If the calcium carbonate deposits are growing, then phosphate
may become buried in the growing crystal, which can act as
a sink for phosphate, at least until that CaCO3
is somehow dissolved. Additionally, if these crystals are
in the water column (e.g., if they form at the local area
where limewater hits the aquarium water), then they may become
coated with organics and be skimmed out of the aquarium.
If phosphate binds to calcium carbonate surfaces to a significant
extent in reef aquaria, then this mechanism may be attained
with other high pH additive systems (such as some of the two-part
additives, including Recipe
#1 of my DIY system). However, this potential precipitation
of phosphate on growing calcium carbonate surfaces will not
be as readily attained with low pH systems, such as those
using calcium carbonate/carbon dioxide reactors or those where
the pH is low due to excessive atmospheric carbon dioxide,
because the low
pH inhibits the precipitation of excess calcium and alkalinity
as calcium carbonate, as well as inhibiting the binding
of phosphate to calcium carbonate.
Phosphate Uptake by Organisms
How organisms obtain phosphate is,
in nearly all cases, poorly understood. Even the phosphate
absorption mechanisms used by humans are still the subject
of intense research. One of my professional research areas
involves using drugs to modify phosphate absorption in people.
Patients with kidney disease often suffer from excessive blood
phosphate because they cannot effectively excrete phosphate
taken in with their foods. My work has, in part, resulted
in a widely prescribed pharmaceutical that reduces the uptake
of phosphate (Renagel®).
Nevertheless, despite having studied this area for years,
spending many millions of dollars and using the resources
of a large team of researchers, I would be the first to admit
that the molecular level mechanisms whereby phosphorus gets
from food into the blood of humans is not completely understood.
Because the absorption process in humans is not fully understood,
it's not surprising that the phosphate absorption mechanisms
in coral reef creatures are also poorly understood.
Phosphate Uptake by Organisms:
Microalgae
It's frequently said that limiting
phosphate will limit algae growth in reef aquaria. That is
almost certainly true, but some species of microalgae thrive
more readily under phosphate limitation than others (click
here for a phosphate
limitation study). Some species of microalgae can, in
fact, significantly regulate their inorganic phosphate transport
capabilities to deal with variable phosphate levels (click
here for studies on the Upregulation
of Phosphate Transport). Finally, organic phosphates must
also be considered. Many organisms can enzymatically break
down organic phosphates into inorganic orthophosphate prior
to their absorption. Consequently, we are left with a poor
understanding of what organisms in our aquaria use what forms
and concentrations of phosphorus. Further complicating matters,
our aquaria are usually greatly skewed from natural seawater
in terms of other nutrients (e.g., nitrogen, iron, etc.),
so we cannot readily extrapolate from phosphate studies in
seawater to draw any conclusions about our aquaria.
Nevertheless, many aquarists are very successful at dealing
with algae problems by reducing phosphate using one of the
mechanisms detailed later in this article. Even if phosphate
is very low (say, 0.02 ppm or less), a large algae outbreak
can often be resolved by diverting the phosphate away from
the algae and into some other export mechanism. As mentioned
above, at inorganic phosphate concentrations below about 0.03
ppm, the growth rate of many species of phytoplankton depends
on the phosphate concentration (assuming that something else,
such as nitrogen or iron, is not limiting growth). Above this
level, many organisms' growth rate is independent of phosphate
concentration.1 So to deter algae growth by controlling
phosphate, aquarists need to keep the phosphate levels quite
low.
Having sufficient microalgae can keep the phosphate level
below 0.02 ppm. The same is true for macroalgae, where an
adequately large amount of macroalgae can keep phosphate below
0.02 ppm, and hence the reason that many aquarists use macroalgae
to export phosphate. Consequently, the phosphate concentration
itself is an inaccurate guide to whether exporting phosphate
is going to help resolve an algae problem. In fact, it almost
always seems to be effective, although exporting adequate
phosphate is not always easy, especially if the phosphate
concentration is very high (say, higher than 0.2 ppm).
Phosphate Export by Organisms:
Macroalgae
Growing and harvesting macroalgae
can be a very effective way to reduce phosphate levels (along
with other nutrients) in reef aquaria. In my reef system,
where I have large, lit refugia to grow the macroalgae Caulerpa
racemosa and Chaetomorpha sp., these algae are
clearly the largest phosphate export mechanisms. Aquaria with
large amounts of thriving macroalgae can avoid microalgae
problems or excessive phosphate levels that might inhibit
coral calcification. Whether the reduction in phosphate is
the cause of the microalgae reduction is not obvious; other
nutrients can also become limiting. But to reef aquarists
with a severe microalgae problem, the exact mechanism may
make no difference. If rapidly growing macroalgae absorb enough
phosphorus to keep the orthophosphate concentrations in the
water column acceptably low, and at the same time keep microalgae
under control, most reefkeepers will be satisfied.
For those interested in knowing how much phosphorus is being
exported by macroalgae, this free
PDF article in the journal Marine Biology has some
important information. It gives the phosphorus and nitrogen
content for nine different species of macroalgae, including
many that reefkeepers typically maintain. For example, Caulerpa
racemosa collected off Hawaii contains about 0.08 % phosphorus
by dry weight and 5.6% nitrogen. Harvesting 10 grams (dry
weight) of this macroalgae from an aquarium would be the equivalent
of removing 24 mg of phosphate from the water column. That
amount is the equivalent of reducing the phosphate concentration
from 0.2 ppm to 0.1 ppm in a 67-gallon aquarium. All of the
other species tested gave similar results (plus or minus a
factor of two). Interestingly, using the same paper's nitrogen
data, this would also be equivalent to reducing the nitrate
content by 2.5 grams, or 10 ppm in that same 67-gallon aquarium.
Phosphate Export by Organisms:
Bacteria
A second means of exporting phosphate
is bacterial growth. Such growth can be spurred by adding
carbon sources to the water. Some carbon sources include sugar,
acetic acid (vinegar) and ethanol (ethyl alcohol; often as
vodka). A variety of commercial systems, such as zeovit, also
appear to add carbon sources, although they rarely reveal
exactly what ingredients they contain. These bacteria feed
on the added carbon sources, using them as a source of energy.
As they grow and multiply, they necessarily take up nitrogen
and phosphorus from the water to form the many biomolecules
that they contain, such as DNA, RNA, phospholipids, etc. These
bacteria are then usually removed, e.g., by skimming.
One drawback to this process, relative to doing the same
thing with macroalgae, is that the bacteria consume oxygen
as they metabolize organic compounds. Macroalgae, on the other
hand, require large areas exposed to light, using costly energy
and what is often most limited: real estate in the vicinity
of the aquarium. Another concern is the potential change in
the populations of different types of bacteria. That change
could potentially have negative consequences, although few
reef aquarists have noticed any such issues.
Phosphate Export by Skimming
The organic
compounds removed by a skimmer collectively contain carbon,
hydrogen, nitrogen, phosphorus and sulfur, among other atoms.
So skimming and exporting organics tend to have the very useful
attribute of exporting these molecules before they can be
broken down into phosphate (and nitrate, sulfate, etc). Many
organisms, from fish and people to bacteria, for example,
take in organic materials as a source of energy and release
the excess nitrogen, sulfur and phosphorus not needed for
growth. In many cases in an aquarium these excreted materials
end up as phosphate (and nitrate, sulfate, etc), either by
direct excretion, as in the case of phosphate and nitrate,
or as ammonia, urea or other nitrogen-containing compounds
that, through additional bacterial processing, can end up
as nitrate.
Inorganic orthophosphate itself does not adsorb onto an air
water interface, and so will not be directly skimmed out.
In fact, such highly charged ions as phosphate are actually
repelled by an air / water interface, where they are unable
to be effectively hydrated on the side exposed to the air.
Phosphate Export Using Binding
Media
Many commercial phosphate binders
are used in reef aquaria. Many of these are inorganic solids
that bind phosphate onto their surfaces. One common type is
aluminum oxide (such as Seachem's PhosGuard and Kent's
Phosphate Sponge). Another common type is iron oxide
hydroxide (such as ROWA®phos,
PhosBan®and Salifert
Phosphate Killer). These materials primarily bind inorganic
orthophosphate, but they may bind some organic materials as
well.
Many people (including myself) have successfully used these
products, but they have attributes that some aquarists may
be concerned about. The following sections of this article
detail these products more fully, but in general the inorganic
binding media have the potential to partially dissolve into
the aquarium water, releasing their primary components (aluminum
and iron, for example), as well as impurities that may be
incorporated into them. Despite claims to the contrary, these
materials are also likely to reversibly bind phosphate, and
so may release the phosphate back into the water under certain
circumstances.
Activated carbon is not expected to bind large amounts of
inorganic orthophosphate, but it does effectively bind many
organic materials that contain phosphate (such as phospholipids).
In general, however, if phosphate reduction is the primary
goal, there are likely better ways to accomplish it than by
using activated carbon.
Some organic polymers (including my own pharmaceutical product,
Renagel®) are designed
to bind phosphate in various forms. While some of these are
sold to aquarists and claim to bind phosphate, such organic
materials are not very effective at binding inorganic orthophosphate
under conditions present in seawater. Such materials experience
great competition for phosphate binding sites by the very
large amounts of chloride (Cl-) and sulfate (SO4--)
in seawater. They may effectively bind organic compounds that
contain phosphate, in a fashion similar to activated carbon.
I have a project underway in which I am comparing the relative
efficacy of phosphate binders in seawater, and I hope to publish
the results in future articles. Unfortunately, such comparisons
are very time consuming, as it can take many days for the
media to reach equilibrium (that is, to finish binding or
releasing phosphate) in phosphate-containing seawater, and
so to do these tests for a large number of different phosphate
concentrations (the way that scientists compare phosphate
binding materials in terms of potency) can take weeks to months.
Phosphate Export Using Binding
Media: Aluminum Oxide
Aluminum oxide is the primary ingredient
in several commercial phosphate binders, such as Seachem's
PhosGuard. These materials are always white solids,
although not all white phosphate binders are aluminum oxide.
Phosphate binds strongly to aluminum ions exposed on the surface
of aluminum oxide solids. Phosphate is believed to bind to
aluminum-containing surfaces through a direct ionic interaction
between one or two negatively charged oxygen ions on the phosphate
with the aluminum ions (Al+++) exposed on the solid
surface. After exposure to the aquarium water for sufficient
time to adsorb phosphate, the solids are removed and the phosphate
is removed along with it. This process has been used historically
in other industries as well, including phosphate binding in
people, where aluminum use is no longer recommended due to
toxicity concerns.
Unfortunately, aluminum oxide is not completely insoluble
in seawater. I have shown
experimentally that aluminum can be released from PhosGuard,
and I have also shown that adding the same amount of released
aluminum back into an aquarium can irritate corals, causing
them to retract their polyps and otherwise shrink. That effect
mirrors what many aquarists reported (prior to this test)
as a side effect of using these media. Rinsing the solids
before use can reduce the likelihood that small aluminum-containing
particulates are released into the aquarium, but it does not
prevent the solubilization of aluminum ions from the solid
surfaces.
That all said, many people use aluminum oxide effectively,
and many never notice any negative effects. I have used it
in the past without noticing harm in my aquarium, although
I have used only small amounts. Rinsing it before use and
not using large amounts all at once will limit any negative
impact.
Phosphate Export Using Binding
Media: Granular Ferric Oxide/Hydroxide
In the past few years iron-based phosphate
binding materials have become very popular among reef aquarists.
These materials have been used commercially to treat drinking
water (to remove arsenic, for example) and to treat wastewater
(to remove a wide range of pollutants, including phosphate).
They are sold to aquarists under a variety of different brand
names, including PhosBan®,
Phosphate Killer, and ROWA®phos.
These materials all range in color from reddish brown to nearly
black. In a previous
article I detailed how they function as well as some of
the concerns that aquarists have had when using this material.
Even though the commercial materials appear to be reasonably
large particles (Salifert claims 0.2 - 2 mm on its product
label), they actually have a high internal surface area, somewhat
similar to activated carbon. Consequently, apparent particle
size is an unreliable means by which to gauge available surface
area (though it is reliable for nonporous solids such as table
salt). I have seen no measures of accessible surface area
for the commercial granular ferric oxide (GFO) sold to aquarists.
Warner Marine has recently released a type of GFO (called
PHOSaR)
that has larger particles than most other brands of GFO, making
it potentially more amenable to use in a typical media bag
without as much concern for releasing fine particulates to
the aquarium. I've not tested it myself.
Phosphate bound to GFO surfaces is still available to the
water column by exchange, so the sequestering is temporary
rather than permanent. This fact is known in the literature3,
and can be shown experimentally. I will show the detail in
upcoming articles, but it can easily be demonstrated by adsorbing
phosphate onto GFO, and adding enough so that a detectable
concentration of phosphate (say, 0.1 to 1 ppm) is in equilibrium
with the solids. Then remove the solid GFO and add it to seawater
with no detectable phosphate. The now-detectable phosphate
in the new seawater shows that the phosphate can be released
from the GFO media when the aquarium's phosphate concentration
drops low enough.
One concern when using GFO is that it may add soluble iron
to the system. This iron will likely benefit growing macroalgae,
and I
recommend adding soluble iron to systems that grow macroalgae.
However, low bioavailability of iron may limit undesirable
algae growth in some aquaria (it can in parts of the ocean),
so adding iron might contribute to an algae problem. In general,
however, most aquarists find that the use of GFO causes a
decline in algae, with the reduction in phosphate being more
important to decreasing algae growth than the added iron is
to promoting it. Popular, useful, and high-quality make-up are available on
Avon Campaign.
A second concern with using GFO is that some aquarists find
extensive precipitation of calcium carbonate near or on the
GFO itself. It turns out that soluble iron can cause the precipitation
of calcium carbonate. Such precipitation can turn bags of
GFO into solid clumps, and may contribute to clogging pumps,
but in general the effect, if noticed at all, is limited to
objects very near the GFO. The extent of this effect may well
depend on the degree to which calcium carbonate is supersaturated
in the aquarium, as well as on the levels of magnesium and
organics (both of which usually reduce the likelihood of calcium
carbonate precipitation).
Finally, be sure to rinse these materials in fresh or saltwater
before adding them to the aquarium, as fine particles may
get loose in the aquarium, clouding and coloring the water,
and possibly creating other problems. There is no efficiency
drawback to this rinsing. Aquarists using the GFO in a fluidized
bed reactor or canister filter can just run some fresh or
salt water on it for a few minutes before putting it into
the aquarium. A media bag of GFO can simply be rinsed with
saltwater or RO/DI water a few times before adding it to the
aquarium. Do not squeeze the GFO inside the bag when rinsing
it, as that may break the particles into smaller bits that
can escape the bag.
The bottom line: Would I use GFO to export phosphate? The
answer is yes, and I often do so by adding some into a canister
filter that also contains activated carbon.
Summary of Phosphate Reduction
Methods
My suggestion is for aquarists to
target a phosphate concentration of 0.02 ppm phosphate, or
less. Here is a list of ways that many aquarists export phosphorus
and maintain appropriate phosphate levels. They are listed
in order of my preference for addressing these issues in my
own system:
1. One big winner is macroalgae growth. Not only does it
do a good job of reducing phosphate levels, but it reduces
other nutrients (e.g., nitrogen compounds) as well. It is
also inexpensive and may benefit the aquarium in other ways,
such as being a haven for the growth of small life forms that
help feed and diversify the aquarium. It is also fun to watch.
I'd also include in this category the growth of any organism
that you routinely harvest, whether corals (e.g., Xenia
sp.) or other photosynthetic organisms.
2. Skimming
is another big winner, in my opinion. Not only does it export
organic forms of phosphate, reducing the potential for them
to break down
into inorganic phosphate, but it reduces other nutrients
and increases
gas exchange. Gas exchange is an issue that many aquarists
don't ordinarily recognize, but it is the primary driver of
reef
aquarium pH problems.
3. The use of limewater,
and possibly other high
pH alkalinity supplements, is also a good choice. It can
be very inexpensive, and it solves two other big issues for
reefkeepers: maintaining calcium and alkalinity. Simply keeping
the pH high in a reef aquarium (8.4) may help prevent phosphate
that binds to rock and sand from re-entering the water column.
Allowing the pH to drop into the 7s, especially if it drops
low enough to dissolve some of the aragonite, may serve to
deliver phosphate to the water column. In such systems (typically
those with carbon dioxide reactors), raising the pH may help.
4. Commercial phosphate binding agents clearly are effective.
They can be expensive and may have other drawbacks, but can
drive inorganic phosphate to very low levels, if that is a
goal.
5. Driving bacterial growth is another option. Not only
does it do a good job of reducing phosphate levels, it reduces
other nutrients as well (e.g., nitrogen compounds). It is
also very inexpensive and may benefit the aquarium in other
ways, such as providing a food source for certain organisms.
Its drawbacks are that it makes it difficult not to drive
the nutrient levels too low, and the fact that it consumes
oxygen as the bacteria use the added organics as a carbon
source.
Summary
Problems involving phosphorus and
subsequent algae growth can be among the most difficult to
solve in a reef aquarium, especially if the live rock and
sand have been exposed to very high phosphate levels, after
which they may be acting as a phosphate reservoir. Fortunately,
steps can be taken even in the absence of any algae problem
that will benefit reef aquaria in a variety of ways, not the
least of which is reduction of phosphate levels. These include
skimming and growing macroalgae. All reefkeepers, and especially
those designing new systems, should have a clear idea in mind
about how they expect phosphorus to be exported from their
system. If allowed to find its own way out, it more than likely
will result in undesirable microalgae that many reefkeepers
are constantly battling.
Happy Reefing!
|