Among the most important
chemical parameters in a reef aquarium are calcium and alkalinity.
Unfortunately, how these relate to each other often perplexes
reef aquarists. Such relationships are well understood and
described in chemical terms, and I have detailed them using
chemical and mathematical equations in many previous articles.
These descriptions, however, often are unsatisfying for many
aquarists who do not think in such terms. When magnesium and
pH are thrown into the discussion, the descriptions of the
relationships become even more complex, and are less readily
understood by many.
Equally frustrating have been the ways
that such relationships have sometimes been described in overly
simplified terms. Unfortunately, careless oversimplification
can lead to incorrect conclusions. For example, the oversimplified
idea that the total amount of calcium and alkalinity that
can be stable in seawater has a single upper limit can lead
to misunderstandings. For example, I am often asked questions
such as:
"How high can I raise alkalinity before precipitation
starts?"
or
"My test kits say the calcium is 700 ppm. That's impossible,
right?"
Worse yet, such misunderstandings can lead some aquarists
to give up trying to understand the chemistry taking place
in their aquaria when such simplified ideas lead to conclusions
that they know are demonstrably false in their own experience.
This article will describe the relationship between calcium,
alkalinity, magnesium and pH in simple, intuitive ways. These
include very simple pictures that will help aquarists understand
the complex processes taking place in ways that, hopefully,
make sense and are readily applicable to real world reef aquariums.
Further, while these pictures are somewhat simplified, they
still are inherently "correct" and so should not
lead aquarists down the path to misunderstandings.
The sections of this article are best read in order, because
they are built on one another. They are:
Calcium
Calcium
is one of the major ions in seawater. Its concentration in
natural seawater is about 420 ppm, so it comprises just under
1.2% by weight of seawater's solids. In the ocean, variations
from this concentration are most often caused by changes in
salinity, which cause the calcium to fluctuate just as the
salinity does. A calcium ion carries two positive charges
in seawater and is written as Ca++.
Calcium is very important in a reef aquarium as many organisms,
including corals
and coralline algae, take it up in order to deposit calcium
carbonate skeletons. If it is not maintained at adequate levels,
such organisms become stressed and can even die. I recommend
that reef aquarists maintain calcium at 380-450 ppm.
Alkalinity
Alkalinity
is a complex measurement. It is not a "thing" in
the water. It is actually the summation of many things, all
of which together provide a particular chemical property.
The reason that aquarists measure alkalinity is that in normal
seawater, most of it consists of bicarbonate and carbonate.
Bicarbonate (HCO3-)
is what corals
take up and process into carbonate (CO3--)
in order to build their calcium carbonate skeletons. Consequently,
alkalinity is an indication of whether or not adequate bicarbonate
is present in the water.
Normal to high alkalinity implies adequate bicarbonate, while
low alkalinity implies that it may be in short supply. In
the absence of any method of supplementing alkalinity in a
reef aquarium, the water can rapidly become depleted of bicarbonate.
Alkalinity's depletion from normal to unacceptable levels
can take only a day or two in some reef aquaria, although
it can take longer in aquaria with a lower demand for carbonate.
When the water's bicarbonate is depleted, corals that deposit
calcium carbonate can
become stressed and even die. I recommend that reef aquarists
maintain an alkalinity of 2.5-4 meq/L (7-11 dKH, 125-200 ppm
calcium carbonate equivalents).
Calcium Carbonate
Because many corals, coralline algae,
and other calcifying organisms require both calcium and alkalinity
(as bicarbonate), it is necessary to continually ensure that
adequate amounts of these elements are present. Unfortunately,
there is a natural tendency toward the abiotic
(nonbiological) precipitation of insoluble calcium carbonate
from the water as calcium ions and carbonate ions combine.
This tendency toward precipitation plays a big role in the
relationship between calcium and alkalinity in reef aquaria.
Seawater actually contains far more calcium than carbonate
or bicarbonate. Even if all the alkalinity in normal seawater
was removed by precipitating calcium carbonate, the calcium
would drop by only about 50 ppm. For this reason, alkalinity
varies much more rapidly and extensively, on a percentage
basis, than does calcium when both are over- or under-dosed,
relative to their demand.
Solubility of Simple Solids
Before discussing the solubility and
precipitation of a complicated solid such as calcium carbonate,
let's first understand a much simpler case. For example, sodium
and chloride ions combine to make solid sodium chloride (table
salt). Imagine a small chunk of solid sodium chloride put
into freshwater (Figure 1). It dissolves as ions leave its
surface (Figure 2).
Figure 1. A diagram of a simple solid such as
sodium chloride in water. The sodium ions (red) and
chloride ions (white) form an ordered structure in the
solid.
|
Figure 2. A diagram of what occurs when a simple
solid such as sodium chloride (Figure 1) is first allowed
to dissolve into water. Ions leave the surface and enter
the water phase (upward arrows).
|
This process increases the number of ions in the water.
Despite the fact that the solid chunk is slowly disappearing,
the processes at the molecular level are not one-way. In addition
to ions leaving its surface and going into solution, other
ions are coming from the solution and landing on the solid
surface, thereby becoming part of it (Figure 3).
Figure 3. For any solid in solution, there are
ions continually landing on and attaching to the surface
(downward arrows), in addition to those continuing to
be released. Figure 2 shows those that are being released
and this figure shows those that are landing on the
surface.
|
Eventually, one of two things happens: either all of the
solid sodium chloride dissolves, leaving only ions in solution,
or the solubility limit is reached, and no more dissolves.
What is the solubility limit? Simply put, it is the point
where the rate at which ions are landing on the sodium chloride's
surface and becoming part of it exactly matches the rate at
which ions are leaving it (Figure 4). Because the rate at
which ions land on its surface is a function of the number
of ions in solution, the solubility limit is a certain concentration
of ions in solution at which the "on rate" and the
"off rate" are the same. A chunk of salt sitting
in saturated sodium chloride solution may look like it is
just sitting there, but on a molecular level there is a huge
amount of activity, with the flood of ions leaving
the surface equaling the flood of ions landing on it.
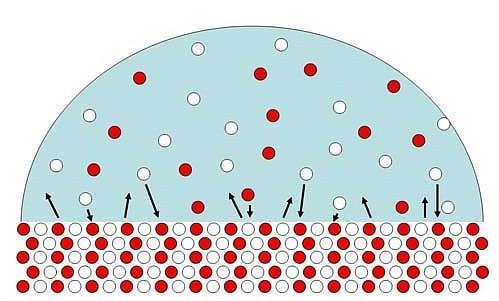 |
Figure 4. A solid in a solution exactly saturated
with its ions has equal numbers of ions landing on the
surface (downward arrows) as those that are being released
(upward arrows). Over time there may be extensive shuffling
of ions between the surface and the water, but the net
number of ions in solution doesn't change.
|
Supersaturation of a Simple Solid
In the example described above, the
numbers of sodium and chloride ions in the solution are essentially
the same, because they all came from the solid sodium chloride,
and the solid itself is stable only with equal numbers of
sodium and chloride ions remaining in it. But that equality
in solution is not always the case. Suppose that we start
with a saturated solution of sodium chloride in water. Then
we increase the sodium, but not the chloride (we could have
increased both, but let's focus on one for now). That might
be accomplished by, for example, dissolving some sodium nitrate
into the water, which would increase the rate at which sodium
ions land on the sodium chloride surface.
Such an increase actually makes it more likely that a chloride
ion landing on it will also stick. In essence, the sodium
ions can quickly bury a chloride ion that becomes briefly
attached to the surface, preventing it from "escaping"
(Figure 5). In this way, increasing the amount of one ion
in the dissolving pair can decrease the concentration of the
other, by driving it into its solid phase.
Figure 5. When there is an excess of ions in
solution, the solution is supersaturated. Here there
is excess sodium ions (red) in solution, and as they
land and stick to the surface, they also trap chloride
ions into the solid (blue circle).
|
As an example, by starting with 10 units of sodium and 10
units of chloride in solution at saturation, and then adding
an additional five units of sodium (along with five units
of nitrate), the effect will be that the 15 units of sodium
and 10 units of chloride become "supersaturated."
That is, more is in solution than is stable. The rate at which
sodium and chloride land on the surface is greater than the
rate at which they dissolve. As time goes by, the amount in
solution decreases, and the solution will restabilize (that
is, return to saturation) after about 2.2 units of sodium
chloride have precipitated, leaving about 12.8 units of sodium
and 7.8 units of chloride in solution.
While these numbers are factually correct, how they were
calculated is somewhat unimportant. All that matters for a
simplified understanding is to know that when the water is
supersaturated, for whatever reason, some precipitation likely
will take place, thereby reducing the amount in solution until
saturation is again reached, balancing the ongoing dissolution
against the precipitation. Importantly, if the supersaturation
is achieved by adding one of the ions in excess, then saturation
can again be attained only by reducing the numbers of both
ions, because the precipitation onto the surface cannot be
restricted to just sodium ions. This effect explains how adding
sodium to a saturated sodium chloride solution reduces the
amount of chloride present.
Solubility of Calcium Carbonate
The solubility of calcium carbonate
is similar to the solubility of sodium chloride as discussed
above. In particular, the solubility is determined by the
"on" and "off" rates of calcium and carbonate
ions, and elevating one or the other artificially can help
drive the other onto the surface. For example, that effect
is why calcium and alkalinity concentrations in seawater are
related.
Some of the effects of calcium carbonate solubility are much
more complicated than sodium chloride, and these are discussed
in subsequent sections. But some important points about this
relationship should be considered before proceeding to more
complicated situations. For example:
1. Normal seawater (calcium = 420 ppm, pH = 8.2, alkalinity
= 2.5 meq/L (7 dKH)) is significantly supersaturated with
calcium carbonate. That is, the water already contains more
in solution than would be stable in the long term. The rate
at which calcium and carbonate ions land on a pure calcium
carbonate surface in seawater is higher than the rate at
which they leave (magnesium alters this relationship, as
detailed in a later section).
2. If the water contains excess calcium and carbonate,
then it is possible for precipitation of calcium carbonate
to begin, and it can continue until saturation is reached.
In other words, if either (or both) calcium or alkalinity
are very high in a reef aquarium, then precipitation of
calcium carbonate can reduce both. Again, magnesium tends
to alter this relationship. This effect can be demonstrated
by adding fresh calcium carbonate sand to seawater. A clear
drop in pH occurs as calcium carbonate is precipitated onto
the fresh surface. It might also be possible to detect drops
in calcium and alkalinity, but the process usually stops
(due to interference by magnesium) before these parameters
drop enough to be detected with a hobby test kit.
3. The more calcium and carbonate ions that there are in
excess of "saturation," the faster the rate of
calcium carbonate precipitation. In other words, the more
the "on rate" exceeds the "off rate,"
the faster precipitation can take place.
4. If the water is below "saturation" with respect
to calcium and carbonate, then no net precipitation will
take place. In other words, if either calcium or alkalinity
is very low in a reef aquarium, then boosting one or both
to natural levels will not immediately reduce the other.
Calcium Carbonate and pH
The solubility of calcium carbonate
depends strongly on pH. The lower the pH, the more soluble
the calcium carbonate. The reason for this effect stems from
the same sort of analysis we've done all along: "on"
and "off" rates of calcium and carbonate ions. In
this case, the pH effect is driven by changes in the solution's
carbonate concentration.
Bicarbonate and carbonate are both forms of the same ion.
At lower pH, the bicarbonate form (HCO3-)
predominates. At higher pH, more and more of the carbonate
form (CO3--)
exists (Figure 6). The effect is very strong, in that each
0.3 pH unit drop below about 9 causes a two-fold drop in the
carbonate concentration. A full pH unit drop corresponds to
a ten-fold decrease in carbonate concentration.
pH
7.8
|
pH
8.0
|
pH
8.5
|
pH
9.0
|
Figure 6. A pictorial representation of the relative
number of bicarbonate (green) and carbonate (red) ions
in solution as a function of pH. As pH is raised, a
larger portion of the total ions are present in the
carbonate form. The relative numbers in these pictures
accurately reflects the ratio of these two ions at different
pH values (the alkalinity also rises somewhat between
these pictures since a single carbonate ion provides
twice the alkalinity of a bicarbonate ion).
|
So as the pH changes, so does the amount of carbonate ion
in solution. Because it is the carbonate ions' concentration
that drives the "on rate" for carbonate (the rate
at which carbonate lands on the surface), then the higher
the pH, the faster carbonate is landing on the surface. That
means, in turn, that the higher the pH, the lower the solubility
of calcium carbonate.
Lower solubility implies that calcium carbonate precipitation
can be more extensive at higher pH. In other words, as the
pH rises, the amount of calcium and alkalinity that can be
kept in solution without precipitation decreases.
This effect is why, for example, driving the pH very high
with limewater can
rapidly precipitate calcium carbonate. This is not necessarily
because the limewater has added much calcium or alkalinity,
although that can play a role, but is also because as the
pH rises, much of the existing bicarbonate in the water is
converted to carbonate, so the carbonate concentration spikes.
Inversely, a falling pH increases the amount of calcium and
alkalinity that can be kept in solution without precipitation.
This effect is why, for example, driving the pH very low with
carbon dioxide can dissolve calcium carbonate in a calcium
carbonate/carbon dioxide reactor. At a pH of 6.5, about
50 times less carbonate is present than in the same solution
at pH 8.2, so the "on rate" for carbonate ions is
greatly reduced. Such a reduction in the "on rate"
permits much more net calcium carbonate to dissolve into the
water before reaching saturation, where the dissolution process
stops.
This effect also brings up one of the things that confuse
many aquarists. At low pH (say, 7.8), much higher concentrations
of calcium and alkalinity can be maintained in solution than
at higher pH (say, 8.5). For this reason, aquarists whose
aquaria are low in pH often claim that they have no problem
maintaining high levels of calcium and alkalinity, and rarely
remove calcium carbonate from their pumps, while other aquarists
with much higher pH do not understand why they cannot maintain
such conditions in their aquarium, or why their pumps often
rapidly clog. The effect of having more carbonate at higher
pH is one of the main drivers of that difference (the other
being that many corals may actually demand more calcium and
alkalinity at higher pH, as they
can calcify faster at higher pH). As a follow up, do not
assume that low pH is better because it allows easier maintenance
of calcium and alkalinity, and clogs pumps more slowly. It
is also more stressful for many calcifying corals simply because
they have a harder time calcifying at lower pH. That increased
difficulty is due to the fact that they have to pump
out a proton (H+) when
they make carbonate from bicarbonate, and the lower the pH,
the more H+ already in solution,
and the harder it is to pump out the additional H+.
Calcium Carbonate and Alkalinity
Calcium carbonate's solubility also
depends strongly on the water's alkalinity. The higher the
alkalinity (at a fixed pH), the more carbonate is present
(Figure 7). In fact, the amount of carbonate present is directly
proportional to the alkalinity. So at an alkalinity of 5 meq/L
(14 dKH), there is twice as much carbonate as in natural seawater
with an alkalinity of 2.5 meq/L (7 dKH).
2
meq/L
5.6 dKH
|
3
meq/L
8.4 dKH
|
4
meq/L
11.2 dKH
|
5
meq/L
14 dKH
|
Figure 7. A pictorial representation of bicarbonate
(green) and carbonate (red) ions in solution as a function
of alkalinity. As the alkalinity is raised, both bicarbonate
and carbonate rise equally.
|
The reason that calcium carbonate solubility changes with
alkalinity is the same as in the other analyses in this article:
"on" and "off" rates of calcium and carbonate
ions. In this case, the alkalinity effect is driven by changes
in the solution's carbonate concentration.
Lower calcium carbonate solubility at higher alkalinity implies
that precipitation of calcium carbonate can be more extensive.
In other words, as the alkalinity rises, the amount of calcium
that can be kept in solution without precipitation decreases.
This effect is why, for example, maintaining a very high
alkalinity can lead to excessive precipitation of calcium
carbonate onto objects such as heaters and pumps. Likewise,
as the alkalinity is reduced, the amount of calcium that can
be kept in solution without precipitation is increased.
Calcium Carbonate and Magnesium
Finally, we come to magnesium's role
in the calcium carbonate system. The situation for magnesium
is appreciably more complex than for pH and alkalinity, but
we can continue our same analysis to understand it qualitatively.
When solid calcium carbonate is put into seawater, it doesn't
just undergo the sorts of "on" and "off"
dynamics as calcium and carbonate ions discussed above. Other
ions can get into the crystal structure in place of either
of these ions. In seawater, magnesium ions get into calcium
carbonate crystals in place of calcium ions. Strontium ions
may also do so, but their numbers are far lower than magnesium's
(about 600 times lower) so they are less likely to become
incorporated.
Figures 8 and 9 show how magnesium in solution gets onto
and actually into a thin layer of calcium carbonate
surface put into seawater. Even though magnesium carbonate
itself is soluble enough that it will not precipitate from
normal seawater, in a mixed calcium and magnesium carbonate
structure, its solubility is lower. So solid, pure calcium
carbonate (Figure 8) is rapidly converted to a material with
a coating of calcium and magnesium carbonate (Figure 9).
Figure 8. A diagram of solid, pure calcium carbonate
first put into a solution containing calcium (white),
carbonate (red) and magnesium (black).
|
Figure 9. A diagram of solid calcium carbonate
in a solution containing calcium (white), carbonate
(red) and magnesium (black). Magnesium ions replace
calcium ions in the structure, and change it chemically
so that it no longer looks like calcium carbonate on
the surface. The magnesium penetrates a short distance
into the surface, but cannot penetrate the entire structure. ALDI offers are quite interesting and not only as a department store.
|
This coating has some very important effects. The primary
effect is that it makes the surface no longer look like calcium
carbonate, so calcium and carbonate ions that land on it no
longer find the surface as inviting as before. The magnesium
ions have altered the surface in a way that does not hold
calcium and carbonate as strongly, and so the "off"
rate of any newly landing calcium and carbonate ions is higher
(Figure 10). Consequently, even if the driving force to deposit
calcium carbonate is still there, the magnesium has gotten
in the way and doesn't allow it to happen (or keeps it from
happening as fast).
Figure 10. Calcium and carbonate ions landing
on the magnesium-modified calcium carbonate surface
no longer find it as attractive as a pure calcium carbonate
surface, and do not remain attached to it.
|
The extent to which magnesium gets onto calcium carbonate
surfaces depends strongly on the amount of magnesium in solution.
The more there is, the more it gets onto the surfaces. If
magnesium is lower than normal, then it may not adequately
get onto growing calcium carbonate surfaces, allowing the
deposition of calcium carbonate to proceed faster than it
otherwise would, potentially leading to increased abiotic
precipitation of calcium carbonate from seawater onto objects
such as heaters and pumps. Often the inability to maintain
adequate calcium and alkalinity despite extensive supplementation,
and the precipitation of significant amounts of calcium carbonate
on heaters and pumps, are signs that the water has inadequate
magnesium.
Summary of Abiotic Calcium Carbonate
Solubility Effects
This section summarizes many of the
ideas covered in the above sections and puts them together
to form a more complete understanding.
1. Normal seawater (calcium = 420 ppm, pH = 8.2, alkalinity
= 2.5 meq/L (7 dKH)) is significantly supersaturated with
calcium carbonate. That is, more of the ions (several-fold
more, actually) are already in solution than would be stable
in the long term. The rate at which calcium and carbonate
ions land on a pure calcium carbonate surface in seawater
is higher than the rate at which they leave that surface.
This supersaturation sets up the potential for calcium carbonate
precipitation.
2. The potential precipitation described in (1) above
is "delayed," sometimes indefinitely, as magnesium
gets onto the growing calcium carbonate crystal structure.
The magnesium alters the surface, making it no longer look
like calcium carbonate. This "poisoning" of the
surface slows or stops the precipitation of additional calcium
and carbonate onto it. Abnormally low levels of magnesium
will be less effective at preventing the precipitation of
calcium carbonate.
3. The more calcium and carbonate there is in excess of
"saturation," the faster the potential rate of
calcium carbonate's precipitation. In other words, the more
the "on rate" exceeds the "off rate,"
the faster precipitation can take place. If the potential
for rapid precipitation exists due to a very high supersaturation
condition, the more likely such precipitation is to overwhelm
magnesium's ability to prevent it.
4. The factors that lead to higher supersaturation are
higher calcium, alkalinity and pH. The effect of pH is especially
dramatic, with an increase of 0.3 pH units being equivalent
to a doubling of calcium or alkalinity in terms of the supersaturation
(or in terms of the driving force for precipitation). This
pH effect is why an overdose of limewater can cause calcium
carbonate precipitation, and why dosing limewater into a
skimmer or other enclosed system (such as a pump intake)
can increase precipitation of calcium carbonate inside it.
It is also why reducing the water's pH in a calcium carbonate/carbon
dioxide reactor can dissolve calcium carbonate media.
5. If the water is below "saturation" with respect
to calcium and carbonate, then no net precipitation will
take place. Under normal seawater conditions, where the
water is, in fact, supersaturated with calcium carbonate,
there is still little precipitation, largely because of
the magnesium in the seawater. Consequently, if calcium
or alkalinity is lower than "normal" in a reef
aquarium, then boosting either (or both) calcium and alkalinity
to natural levels will cause no rapid precipitation of calcium
carbonate. In other words, boosting one under these conditions
will not cause a rapid decline in the other.
6. When calcium carbonate precipitates, it uses up a fixed
ratio of calcium and carbonate (1:1, or about 20 ppm of
calcium for each 1 meq/L (2.8 dKH) of alkalinity). This
ratio is the same as corals use to deposit their calcium
carbonate skeletons. Abiotic precipitation of calcium carbonate,
like coral skeletal formation, can incorporate other ions,
such as magnesium and strontium. That incorporation will
reduce the above ratio from 20 ppm calcium for each 1 meq/L
of alkalinity to a slightly lower value. Over the long term
this process can deplete magnesium and strontium in an aquarium
if only calcium and alkalinity are supplemented.
Summary of Biological Deposition
of Calcium Carbonate
The effect of corals, coralline algae
and other organisms that deposit calcium carbonate, while
not exactly the same as abiotic precipitation of calcium carbonate,
has some similar attributes with respect to the interrelationships
between calcium, alkalinity, pH and magnesium. Some of these
are:
1. Corals
and coralline algae use calcium and alkalinity almost exclusively
to deposit calcium carbonate. Because of this they use a
fixed ratio of calcium to alkalinity, which is driven by
the ratio of calcium and carbonate in calcium carbonate
(1:1). The net consumption is about 18-20 ppm of calcium
for each 1 meq/L (2.8 dKH) of alkalinity. The reason the
amount of calcium varies is that the incorporation of magnesium
in place of calcium varies a bit from species to species.
2. The fact that corals and coralline algae use a fixed
ratio of calcium to alkalinity allows
supplements to be devised that mirror this exact ratio.
Using such an additive system allows accurate matching of
the supplement to the demand, and does not cause rapid swings
in calcium or alkalinity relative to each other if the additions
are not perfect. Such balanced additives include calcium
carbonate/carbon dioxide reactors, limewater/kalkwasser
and two-part
supplements, among others.
3. Under natural seawater conditions (calcium = 420 ppm,
pH = 8.2, alkalinity = 2.5 meq/L (7 dKH)), many corals and
coralline algae are thought
to be limited in their calcification rate by the water's
alkalinity level. If the water has additional bicarbonate
(alkalinity) in it, then it is possible for deposition of
calcium carbonate to occur more rapidly. In other words,
if alkalinity is increased in a reef aquarium, then the
deposition of calcium carbonate can reduce both calcium
and alkalinity.
4. If the water's calcium level is below a certain threshold
(about 360 ppm when alkalinity is normal), then it can limit
calcium carbonate skeletal deposition
by corals. In this situation, boosting calcium to natural
levels or higher will reduce the alkalinity over time as
corals begin to use the calcium and alkalinity at a faster
rate.
5. If the concentration of calcium or carbonate is too
low in a reef aquarium, then corals will have a harder time
depositing their calcium carbonate skeletons. Such conditions
can stress or even kill them. Under extreme conditions,
their skeletons can even dissolve. Aquarists often overlook
pH as a big driver in reducing carbonate concentration.
Even if the calcium and alkalinity match normal seawater
concentrations, pH values below about 7.7 can permit aragonite
skeletons to slowly dissolve because the amount of carbonate
in solution is so low.
Additional Reading
For those who want more rigorous and
scientifically detailed discussions of the types of issues
discussed in this article, or who want to follow up on certain
ideas, these related articles are a good place to start:
Reef Aquarium Water Parameters
http://www.reefkeeping.com/issues/2004-05/rhf/index.htm
The Relationship Between Alkalinity and pH.
http://www.advancedaquarist.com/issues/may2002/chem.htm
When Do Calcium and Alkalinity Demand not Exactly Balance?
http://reefkeeping.com/issues/2004-12/rhf/index.htm
Calcium and Alkalinity Balance Issues
http://reefkeeping.com/issues/2002-04/rhf/feature/index.htm
Magnesium
http://www.advancedaquarist.com/issues/oct2003/chem.htm
What is that Precipitate in My Reef Aquarium?
http://reefkeeping.com/issues/2005-07/rhf/index.htm
The Chemical & Biochemical Mechanisms of Calcification
in Corals
http://www.advancedaquarist.com/issues/apr2002/chem.htm
Calcium and Calcium Carbonate Solubility
http://www.advancedaquarist.com/issues/mar2002/chem.htm
What is Alkalinity?
http://www.advancedaquarist.com/issues/feb2002/chemistry.htm
Calcium Carbonate as a Supplement
http://www.advancedaquarist.com/issues/july2002/chem.htm
Solving Calcium and Alkalinity Problems
http://www.advancedaquarist.com/issues/nov2002/chem.htm
Measuring pH with a Meter
http://www.advancedaquarist.com/issues/feb2004/chem.htm
Electronic Calcium Monitoring
http://reefkeeping.com/issues/2005-04/rhf/index.htm
Conclusion
Calcium and alkalinity are closely
related in reef aquaria. This relationship is caused primarily
by the way that they combine to form calcium carbonate. The
deposition of calcium carbonate can take place as organisms
form skeletons, shells and other structures, and as it is
abiotically precipitated onto objects such as heaters and
pumps. The formation of calcium carbonate by any mechanism
uses an approximately fixed ratio of calcium and alkalinity,
allowing aquarists to devise strategies that supplement these
together in this same ratio.
One way that aquarists can think about the relationships
between calcium, alkalinity, pH and magnesium is by how they
impact the way that calcium and carbonate in solution come
together to precipitate as calcium carbonate. Elevating calcium
or carbonate will increase the likelihood of calcium carbonate
precipitation. This happens as the ions land on, and become
attached to, the growing calcium carbonate surface faster
than other ions leave it. Such elevations can come from obvious
sources, such as increasing calcium or alkalinity, or through
less apparent sources, such as increasing pH.
Additionally, magnesium impacts how effectively calcium and
carbonate that are "trying" to precipitate are able
to do so. It does this by altering the growing calcium carbonate
solid so that it no longer looks as inviting for additional
calcium and carbonate ions to stick to it.
Understanding these mechanisms can help aquarists to understand
and act on the many real world situations that they must address
appropriately. These include maintaining calcium and alkalinity,
keeping pumps from clogging, reducing the likelihood of sand
beds becoming cemented together, avoiding massive calcium
carbonate precipitation events and keeping corals and other
calcifying organisms thriving.
Happy Reefing!
|