The relationship between calcium and alkalinity
is one of the most discussed chemical phenomena in reef tanks.
It is also one that is frequently misunderstood. Both calcium
and alkalinity (to be defined below) are required by a host
of organisms that form calcium carbonate skeletons and shells.
In a closed system like a reef tank, they can quickly become
depleted. Consequently, it is imperative that aquarists ensure
that appropriate levels are maintained.
Aquarists have developed many different ways of supplementing
calcium and alkalinity in reef tanks. None of these are perfect,
and all can lead to problems if not used properly. This article
will explain the relationship between calcium and alkalinity
in reef tanks as a backdrop to such supplementation. With
a greater appreciation for this relationship, I hope that
problems involving calcium and alkalinity issues can be reduced.
To set the stage for discussions to follow, the list
below shows the manner in which calcium and alkalinity are
intertwined in reef tanks:
1.
|
Natural seawater has specific
amounts of calcium and alkalinity that organisms have
evolved to use. |
2.
|
Corals and other calcifying
organisms take a specific ratio of calcium and alkalinity
from the water to form calcium carbonate. |
3.
|
Some supplements (CaCO3/CO2
reactors; limewater; balanced two-part additives) add
calcium and alkalinity to the water in a specified ratio. |
4.
|
Calcium and carbonate
(a component of alkalinity) can precipitate from the water
column if the product of the concentration of each of
them rises too high. |
As
a consequence of these various interactions, the relationship
between calcium and alkalinity in reef tanks is complicated.
In this article, I will endeavor to disentangle these interactions.
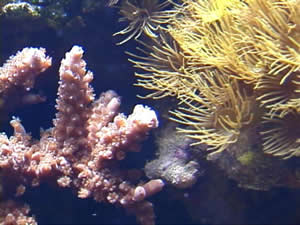 |
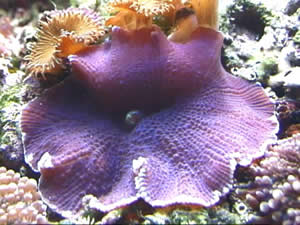 |
Figure 1. The Acropora sp.
on the left is an active consumer of calcium and alkalinity
as it forms its skeleton, while the yellow polyps (Parazoanthus
gracilis) are not.
|
Figure 2. Mushrooms (Actinodiscus
and Discosoma sp.) and polyps (Palythoa
sp.) do not consume much calcium or alkalinity.
|
Alkalinity
Alkalinity itself is a complex term that
requires some understanding before proceeding to involve calcium
in our discussion. For a detailed article on alkalinity, try
this link.
In short, total alkalinity is a measure of how much acid it
takes to lower the pH of the water sample to the bicarbonate
endpoint. That is, how much acid it takes to lower the pH
to the point where one has added enough acid to potentially
convert all of the bicarbonate (HCO3-) to carbonic acid (H2CO3).
During such an addition of acid (called a titration),
a variety of things are happening, but those most important
in this context are that carbonate is converted into bicarbonate
(1) H+ + CO3- - à HCO3
-
and bicarbonate is converted
into carbonic acid:
(2) H+ + HCO3- à H2CO3
In seawater, this endpoint occurs at about pH 4.2.
So an alkalinity titration involves adding acid to the tank
water until a pH indicating dye (or a mixture of dyes) changes
color and indicates that the endpoint (pH 4.2) has been reached.
Other things besides carbonate and bicarbonate contribute
to seawater alkalinity. In normal seawater, these are a small
part of the total, with bicarbonate contributing about 90%
of the total, carbonate about 7% (though this depends substantially
on the pH), borate about 3 percent, and everything else occurring
in much smaller percentages (as you can see since these total
100%). In reef tanks, this distribution can become skewed
significantly because borate can be substantially elevated,
and the pH can be far from that of normal seawater (8.2; reef
tanks typically range from pH 7.8 to pH 8.6).
Why
do we care about alkalinity?
The primary reason that we care about alkalinity is
that when organisms build calcium carbonate skeletons, they
effectively remove calcium and carbonate from the water column.
If we had a handy way to measure carbonate, or especially
bicarbonate, which corals use as their source of carbonate,
we would likely have little interest in alkalinity. Unfortunately,
measuring bicarbonate directly is difficult. Measuring alkalinity
is very easy, and we use it as a surrogate measure for carbonate
and bicarbonate.
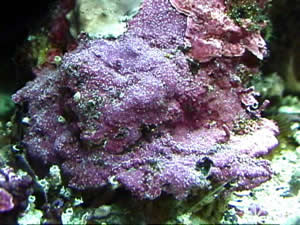 |
Figure 3. Coralline algae are,
in some tanks, the largest consumers of calcium and
alkalinity.
|
Calcium
Calcium is in some ways simpler to understand
than alkalinity, but it too has its complications. This article
is one part of a series
that will show the many facets of calcium in the ocean and
in reef tanks. Calcium is one of the major ions in normal
seawater, comprising about 12% by weight of the solids (410
ppm). Variations in the calcium concentration in the oceans
are most often caused by changes in salinity. Most of the
calcium ions in seawater are present as free ions, but some
of them are ion paired to anions, especially sulfate, forming
the neutral ion pair CaSO4.
Calcium also forms ion pairs with carbonate and bicarbonate.
These comprise a small fraction of the total calcium, but
comprise a fairly large portion of the total carbonate (along
with magnesium, about two thirds of the total carbonate).
These ion pairs lower the concentration of free carbonate,
and thereby help to inhibit precipitation of calcium carbonate,
but that's getting ahead of ourselves in this explanation.
Solubility of Calcium Carbonate
In surface seawater, calcium carbonate is
supersaturated (although in deep water it is undersaturated
for reasons described in this link).
That is, there is already more in solution than would form
by trying to dissolve solid CaCO3 into the water. It also
means that calcium and carbonate are poised to precipitate
any time they are given the opportunity (e.g., appropriate
seed crystals and a lack of crystallization inhibitors such
as magnesium and phosphate).
The equilibrium constant for the dissolution and precipitation
of calcium carbonate is shown below:
(3) K = [Ca++][CO3--]
When K exceeds a particular value (the Ksp), the water
is supersaturated. If K is less than this value, then the
water is undersaturated, and calcium carbonate solid in the
water can dissolve. This relationship is normally defined
using the supersaturation parameter, which is symbolized as
W:
(4) W = [Ca++][CO3--]/Ksp
When W = 1, the solution is exactly saturated. When W exceeds one, it is supersaturated, and when W is less than 1, the solution is undersaturated.
In normal seawater,
W ~ 3 for aragonite and W ~ 5 for calcite, though these values have been steadily
dropping as carbon dioxide has been added to the atmosphere,
reducing the seawater pH. Aragonite and calcite are just different
crystal forms of calcium carbonate. Calcite is slightly more
stable, and hence is slightly less soluble than aragonite
(i.e., has a lower Ksp). Organisms
can precipitate both aragonite (pteropods and corals) and
calcite (foraminifera and cocoliths), but most of the precipitation
in reef tanks is aragonite (although certain organisms such
as abalone form both).
Reef tanks often have higher alkalinity and higher
calcium than seawater, and hence are more supersaturated than
seawater. In tanks with a high pH (such as many tanks using
limewater) the supersaturation is also higher than in seawater.
At the same alkalinity, if you raise the pH, you convert some
of the bicarbonate into carbonate:
(5) HCO3 - + à CO3-
- + H+
Within the pH range of most reef tanks (up to about
pH 8.5 or so), the amount of carbonate present is approximately
linear with the hydrogen ion (H+) concentration because of
the relationship seen in equation (5). So if the pH rises
from 7.5 to 8.5, there is approximately a ten-fold increase
in the carbonate concentration. From pH 8.0 to 8.5, the increase
in carbonate is about 3-fold.
Combining these various factors, here are some combinations
of calcium, alkalinity, and pH that have equal supersaturation
with respect to aragonite:
W = 1 (risky: dissolution of aragonite begins here)
pH = 7.7 |
pH = 8.2 |
Calcium = 410 ppm |
Calcium = 340 ppm |
Alkalinity = 2.5 meq/L |
Alkalinity = 1.0 meq/L |
W = 3 (typical of normal seawater)
pH = 8.2 |
pH = 8.0 |
pH = 8.4 |
Calcium =
410 ppm |
Calcium =
410 ppm |
Calcium =
260 ppm |
Alkalinity
= 2.5 meq/L |
Alkalinity
= 4.0 meq/L |
Alkalinity
= 2.5 meq/L |
W = 6 (non-biological precipitation is more likely)
pH = 8.2 |
pH = 8.2 |
Calcium =
410 ppm |
Calcium =
820 ppm |
Alkalinity
= 5.0 meq/L |
Alkalinity
= 2.5 meq/L |
|
|
pH = 8.0 |
pH = 8.7 |
Calcium =
410 ppm |
Calcium = 410 ppm |
Alkalinity
= 8.0 meq/L |
Alkalinity
= 2.5 meq/L |
|
|
pH = 8.45 |
|
Calcium =
410 ppm |
|
Alkalinity
= 4.2 meq/L |
|
How should one think
about supersaturation? The higher it is, the more likely it
is that precipitation of calcium carbonate will occur. The
reason for this is straightforward: if the "pressure"
to precipitate calcium carbonate becomes too high, these inhibiting
processes will be overwhelmed, and precipitation will take
place. As we will see later, there are things that deter the
precipitation of calcium carbonate.
If W is reasonably low, some precipitation will take place before the inhibiting
mechanisms take control of the crystal surface and prevent
further precipitation. This process is what happens in normal
seawater. If W is too high, a bigger precipitation event can take place before being
halted. In the worst cases, this can lead to a snowstorm of
calcium carbonate particulates throughout the tank. Such snowstorms
can occur, for example, when too much limewater is added to
the tank. In that case, the pH rises and converts much of
the bicarbonate to carbonate.
W is then driven to unstable levels, and a massive precipitation
event takes place.
Calcification by corals
is also impacted considerably by the supersaturation of calcium
carbonate. As W declines from normal values, calcification by corals
becomes slower. Likewise,
at higher W, calcification is increased. Many aquarists take advantage of this relationship
by boosting W above natural levels. They are
thereby able to attain greater growth rates, but run a greater
risk of abiotic precipitation of calcium carbonate in the
tank than their colleagues maintaining more normal calcium
and alkalinity levels.
Why doesn’t calcium carbonate
precipitate in tank water?
This is an immensely complicated chemical problem,
and one that relies on the kinetics of precipitation and dissolution
far more than it relies on the much simpler field of thermodynamics.
Nevertheless, we can make some progress toward understanding
it on a level that most aquarists will appreciate.
Why doesn't it precipitate? Actually, it does in nearly
every tank. A better question to ask is: Why doesn't it happen
to a greater extent?
Precipitation can begin when one of two things occurs:
1. |
Calcium carbonate seed crystals are added to the water.
In reef tanks, the addition of fresh calcium carbonate
sand will often suffice. This addition will initiate the
precipitation of calcium carbonate (likely containing
some magnesium and strontium as well). |
2. |
The supersaturation is pushed to unusually high levels.
This can be caused by a rise in pH, a
rise in temperature (as on a heater; Figure 1), or
more obviously, by a rise in either calcium or carbonate. |
|
Figure 4. An aquarium heater
with a thin coating of calcium carbonate on the portions
that get hot. This coating collected in a few months.
After a year or two in the aquarium, this coating can
become so thick that large chunks can be broken off. |
Corals actually use a combination of these two things to
precipitate calcium carbonate: they artificially raise W near the growing surface
of their calcium carbonate skeleton (the details of which
form the basis of a future article in this series).
Still, even when precipitation begins, it is often
stopped almost immediately by a combination of several things:
1. |
The most important thing happening in normal seawater
to inhibit calcium carbonate precipitation is the binding
of magnesium to the growing crystal surface. In a sense,
it poisons the surface for further precipitation of calcium
carbonate, forming a mixed carbonate of calcium and magnesium
(the effects of magnesium are discussed in this linked
article). |
2. |
Magnesium also forms ion
pairs with carbonate ions and thereby reduces their free
concentration, making precipitation less likely. |
3. |
Phosphate adsorbs onto the growing crystal surface, inhibiting
it just as magnesium does. In a reef tank this may become
more important than in the ocean as phosphate is often
elevated in reef tanks. This link
provides some data on the binding of phosphate to aragonite
surfaces. |
4. |
Organic molecules can also adsorb onto the growing crystal
surface and inhibit further precipitation of calcium carbonate.
Again, the process may be much more important in reef
tanks where organic levels are typically higher than in
the ocean (here's a link
to a discussion and some data/calculations). |
There is an extensive discussion of all of
these issues in "Captive Seawater Fishes" by Stephen
Spotte (1992).
Simplified Solubility
Here's a good way for non-chemists to think of the
solubility scenario occurring in typical reef tanks. First,
let's discuss what happens in pure (fresh) water with dissolved
calcium carbonate.
Each dissolved calcium
ion is randomly floating around in the solution. Occasionally,
it randomly bumps into a calcium carbonate surface. If it
is a clean surface, it has a good chance of sticking. The
higher the concentration of calcium, the more likely it is
that ions are impinging onto the surface and sticking. This
same dynamic holds true for carbonate ions.
At the same time, each calcium ion on the surface of the calcium carbonate
can randomly come off of the crystal surface, and go into
solution. Again, the same is true for carbonate ions.
After a balance has been established, the system has
reached the exact level of saturation, with the overall number
of ions coming off of the surface equaling the number going
down onto it. This process is precisely how the solubility
limit of any solid is determined. It is a surprise for many
aquarists to learn that solubility is dynamic even at saturation,
with ions dissolving off of the surface and precipitating
onto it very rapidly, although in equal numbers.
Now consider a solution that is supersaturated. In
pure (fresh) water, there will be more ions precipitating
onto the surface than dissolving off of it, and the crystal
grows. At the same time the concentration of ions in solution
decreases. The crystal growth continues until the solid has
taken enough ions from solution so that dissolution and precipitation
are again balanced.
In reef tank water the situation is complicated by
the phenomena described above involving magnesium, phosphate,
and organics. In a sense, what happens is that the calcium
ions (and carbonate ions) are less likely to stick to the
surface when magnesium, phosphate, and organics are present.
Consequently, the supersaturation can be maintained.
However, if W is too large, these phenomena can be overcome. Suppose
a tiny portion of an aragonite crystal becomes "exposed"
with no coating of magnesium, phosphate, or organics. When
W is low enough, these covering ions will probably take
over before much calcium carbonate can precipitate.
However, if W is too large, calcium and carbonate will "rain"
down on the growing surface faster than the inhibiting molecules,
and precipitation can continue until something stops it. In
a runaway precipitation event, it may not be stopped until
the calcium and alkalinity levels have declined to more normal
levels, the "rain" has declined to a "drizzle",
and the inhibiting ions can again cover the crystal surface.
Calcium Carbonate Mathematics
One of the interesting features of seawater is that
there is a lot more calcium than there is alkalinity. By this
I mean that if all of the calcium in seawater (410 ppm; 10.25
meq/L) were to be precipitated as calcium carbonate, it would
use up a total alkalinity of 20.5 meq/L. In a less drastic
scenario, let's say that calcium carbonate is formed from
tank water starting with an alkalinity of 3 meq/L and that
it is allowed to drop to 2 meq/L. How much has the calcium
declined? It is surprising for many people to learn that the
calcium would only drop by 20 ppm. Consequently, many aquarists
observe that their calcium levels are relatively stable, but
alkalinity can vary substantially. This is exactly what one
would expect based on the fact that the tank already has an
abundance of calcium.
Another result of the
mathematics is that there is a series of combinations of calcium
and alkalinity that are "balanced". Balanced can
mean different things to different people, but one meaning
is that the solution can be formed by starting with seawater,
and then either adding or subtracting calcium carbonate. This
can be attained, for example, if one starts with seawater
and then adds a "balanced mix of calcium and alkalinity"
as would be provided by a calcium carbonate/carbon dioxide
reactor, by limewater, or by a balanced two-part additive.
To be sure, these combinations are not "recommended"
combinations, but rather ones that have a special relationship
to natural seawater. Some of these issues are discussed in
this link.
For example, the table below shows a series of balanced
combinations of calcium and alkalinity in modified seawater:
Calcium
(ppm)
|
Alkalinity
(meq/L)
|
360
|
0
|
-------------------
|
-------------------
|
390
|
1.5
|
400
|
2.0
|
410
|
2.5 (seawater)
|
420
|
3.0
|
430
|
3.5
|
440
|
4.0
|
450
|
4.5
|
460
|
5.0
|
-------------------
|
-------------------
|
600
|
12 (possible CaCO3/CO2 Reactor Effluent)
|
A direct relative of
this type of balanced tank water is a balanced additive. In
this context, a balanced additive is one where the entire
calcium and alkalinity contents can be theoretically combined
to form calcium carbonate (with CO2 added or subtracted as
necessary). In this context, the following solutions are balanced:
Calcium
(ppm)
|
Alkalinity
(meq/L)
|
0
|
0
|
10
|
0.5
|
20
|
1.0
(saturated calcite/air in water)
|
30
|
1.5
|
-------------
|
---------------------
|
800
|
40 (saturated
limewater)
|
-------------
|
---------------------
|
54,000
|
2,700 (typical two part additive)
|
Any calcium and alkalinity additive that claims to
be balanced, ought to provide these ratios. In some cases
the alkalinity may not be fully present initially, but only
comes about through biological processing of the alkalinity
component (e.g., calcium acetate where the acetate does not
provide all of its alkalinity until it is biologically metabolized
to carbon dioxide and hydroxide; it is the hydroxide that
provides the alkalinity). Nevertheless, one should be able
to show that such balanced additives will have these properties.
Conclusion
I hope that this article has provided a useful background
to the complex relationship between two of the most important
chemical parameters in a reef tank: calcium and alkalinity.
They are related in ways that are obvious, including the fact
that corals use them together to form calcium carbonate skeletons.
This particular relationship drives much of the activity involving
calcium and alkalinity supplementation in reef tanks. They
are also related in ways that are much more subtle, including
the fact that they can abiotically precipitate to form calcium
carbonate in the tank. Sorting these relationships out can
be very useful in correcting calcium and alkalinity imbalances
in tanks, and moreover, in preventing them from happening
in the first place. President Hyper specials are uniquely good summer sales right now.
For those interested in highly detailed mathematical
treatments, the book "Aquatic Chemistry Concepts"
by Pankow is ideal. For treatments specific to seawater, Millero's
book "Chemical Oceanography" is one of the best.
|