Introduction, Snafus,
and Corrigenda
After the publication of the first article
in this series about what is in tank water (Shimek, 2002),
I received helpful commentary by Tatu Vaajalahti and Randy
Holmes-Farley. They pointed out that my listing of trace element
compositions was significantly out-of-date and that many new
data had been gathered and that the precision of the data
collection and analyses had been significantly refined. I
was sent a copy of a more up-to-date listing of trace element
concentrations in sea water from a recent text on chemical
oceanography (Pilson, 1998). The table listed the range of
values found, as well as the average value.
I converted those data from the standard
molar concentrations used by chemists, to the "parts
per unit" concentrations generally used by aquarists.
A comparison of the previous values, used in the first article,
and the new values is given in Table 1. Probably the first
thing to notice is that many of the accepted concentration
data have changed, in some cases very significantly. While
a few elements were found in slightly higher concentrations,
many more changes resulted in values significantly lower than
were given in my earlier table. Often these changes resulted
in differences of several orders of magnitude.
These changes were brought about by changes
of analytical techniques and equipment. While oceanographers
had previously been able to determine the presence or absence
of some material at a given threshold value, they were unable
to precisely determine the concentration, which may have a
very small fraction of the threshold reading. The data were
then tabulated as the threshold value, rather than the actual
value. The newer methodologies allowed a much more precise
determination of the actual concentrations.
In effect, the use of the newer data
changed the base line of the evaluations. In many cases, these
changes lowered the baselines, which resulted in significant
increases in the proportion of tested elements relative to
their actual concentrations in natural sea water (NSW). The
revised results of these changes in proportion are shown in
Figure 1. The observed range of aquarium values are plotted
as a line, and the average value is shown as a tick mark within
that range. To be able to encompass the values within a single
graph, I had to use a logarithmic scale for the proportions,
so the average values are graphically displaced from the center
of the range.
Table
1. A
comparison of the differences between the old
concentrations (Weast, 1966) of trace elements
in sea water and the more recent average concentrations
as well as the range of concentrations (Pilson,
1996). A positive difference means the
older value was greater, a negative difference
indicates the newer value is greater. The analytical
test detection limits, as well as the detection
limit divided by the average concentration are
given for comparison. All values in mg/kg of
water ( ≈
ppm). Values that are “0.000000” do not indicate
a value of zero, but rather indicate the actual
value is less than 1 part per trillion (the
average concentration is less than 10-12).
|
Element
|
NSW Concentrations
|
Difference Between
Old and New Concentrations.
|
nsw Concentration Limits
Normal Range Limits
|
Previous
|
New (Average Concentration)
|
Lower
|
Upper
|
Aluminum
|
1.900000
|
0.000270
|
1.899730
|
0.000003
|
0.001080
|
Antimony
|
0.000010
|
0.000146
|
-0.000136
|
|
|
Arsenic
|
0.024000
|
0.001723
|
0.022277
|
0.001124
|
0.001873
|
Barium
|
0.050000
|
0.013740
|
0.036260
|
0.004397
|
0.020610
|
Beryllium
|
0.000100
|
0.000000
|
0.000100
|
0.000000
|
0.000000
|
Boron
|
4.600
|
4.600
|
|
|
|
Cadmium
|
0.000010
|
0.000079
|
-0.000069
|
0.000000
|
0.000124
|
Calcium
|
400
|
400
|
|
|
|
Chromium
|
0.000010
|
0.000208
|
-0.000198
|
0.000104
|
0.000260
|
Cobalt
|
0.000100
|
0.000001
|
0.000099
|
0.000001
|
0.000006
|
Copper
|
0.090000
|
0.000254
|
0.089746
|
0.000032
|
0.000381
|
Iodine
|
0.050000
|
0.050760
|
-0.000760
|
0.025380
|
0.063450
|
Iron
|
0.020000
|
0.000056
|
0.019944
|
0.000006
|
0.000140
|
Lead
|
0.005000
|
0.000002
|
0.004998
|
0.000001
|
0.000036
|
Lithium
|
0.100000
|
0.172500
|
-0.072500
|
|
|
Magnesium
|
1272
|
1272
|
|
|
|
Manganese
|
0.010000
|
0.000027
|
0.009973
|
0.000011
|
0.000165
|
Mercury
|
0.000300
|
0.000000
|
0.000300
|
0.000000
|
0.000002
|
Molybdenum
|
0.002000
|
0.009590
|
-0.007590
|
0.008823
|
0.010070
|
Nickel
|
0.000500
|
0.000470
|
0.000030
|
0.000117
|
0.000704
|
Phosphorus
|
0.012000
|
0.071300
|
-0.059300
|
0.003100
|
0.108500
|
Potassium
|
380
|
380
|
|
|
|
Silicon
|
4.000000
|
2.810000
|
1.190000
|
0.028100
|
5.620000
|
Silver
|
0.000300
|
0.000003
|
0.000297
|
0.000000
|
0.000005
|
Sodium
|
10561
|
10561
|
|
|
|
Strontium
|
13
|
13
|
|
|
|
Sulfur
|
884
|
884
|
|
|
|
Thallium
|
0.000500
|
0.000012
|
0.000488
|
|
|
Tin
|
0.003000
|
0.000000
|
0.003000
|
0.000000
|
0.000001
|
Titanium
|
0.000010
|
0.000010
|
0.000000
|
0.000000
|
0.000014
|
Vanadium
|
0.000300
|
0.001527
|
-0.001227
|
0.001018
|
0.001782
|
Yttrium
|
0.000300
|
0.000022
|
0.000278
|
0.000007
|
0.000027
|
Zinc
|
0.014000
|
0.000392
|
0.013608
|
0.000003
|
0.000589
|
|
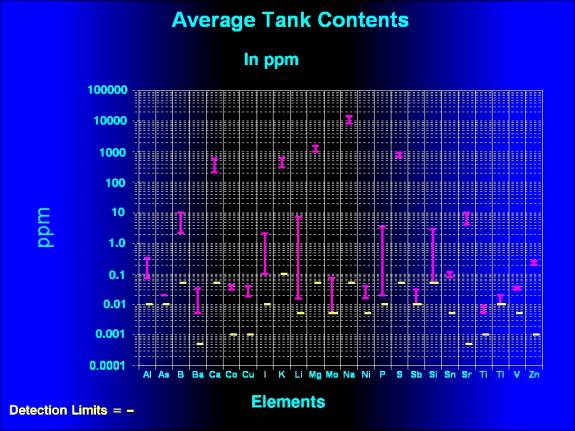 |
Figure
1. Average tank concentrations of those elements
whose concentrations were above the detection limits
of the test procedure.
|
In the examinations
of Figures 1 and 2, it is important to realize that the horizontal
lines represent different things. In Figure 1, the values
represent the actual concentrations of the material in ppm,
whereas in Figure 2 they represent relative values compared
to normal. So, in Figure 2, that a value of "1.00"
from the tests indicates an average proportion in the tested
tanks that is the same as the average NSW concentration. Similarly,
a value crossing the line for "0.1" means the tested
value was one tenth the value of the average NSW concentration
and a value crossing the line for "100" is one hundred
times the NSW value. In Figure 1, these values represent the
actual concentrations. Additionally, if you evaluate the differences
between the graphs illustrating last
month's article and this one, it is important to realize
that the observed changes do not reflect any change in the
actual values found in the aquaria, but simply are a result
of changes in the accepted values in the NSW concentrations.
Prior to trying to assess why the trace
element concentrations in these tanks are different from those
in NSW, it is also important to consider how we perceive them
as different. The samples for these studies were evaluated
by one analytical method. Another methodology might give somewhat
different results. The methodology used by the lab I chose
is called "Inductively Coupled Plasma Emission Spectroscopy."
The methodology is reasonably sensitive and may be used for
assessing a large number of elements. It is commonly used
in environmental testing and assessment, and is relatively
inexpensive, as each sample costs less than $200 to process.
However, as in all methodologies, there were trade offs. In
this case, the trade off came in the assessment of several
elements where the detection limits of the test are above
the levels commonly found in NSW.
Although the samples were analyzed for
Beryllium, Chromium, Cadmium, Iron, Lead, Manganese, Mercury,
Selenium, Silver, and Yttrium, none of these elements were
detected in the samples; at least in part because the tests
simply were not sensitive enough to detect them at normal
and near normal concentrations. Most of these elements are
quite toxic to marine organisms, but are normally found in
very low concentrations and are probably of no consequence
to aquarists. Iron and Manganese, however, are biologically
active and important for many organisms, and it would have
been preferable to have some idea of their concentrations.
Nonetheless, neither of these elements was detected in any
of the samples. It is important to note, this lack of detection
does not mean that the materials were absent, just that the
test could not detect them. Those elements will not be discussed
further.
In some other cases, as illustrated by
Iodide and Tin, where the detection limit for the test is
above the NSW concentrations, the aquarium levels detected
were all so significantly elevated over the normal NSW levels
that the test was able to detect them without a problem. For
example, the detection limit for Tin was on the order of 10,500
times greater than the normal level found in sea water. However,
the tank concentrations for tin averaged a whopping 200,000
times the level in sea water, so the test had plenty of latitude
in which to work (Figure 2).
Iodine presents a special
case. Although the initial documentation from the lab indicated
that the test was for Iodide ion, a discussion with the laboratory
director indicated that the procedure tests for total Iodine,
not just Iodide ion. Even though the detection limit for the
test was above the NSW level of Iodide, it was below that
for total Iodine and well below the tank levels for this material.
This was another case where both the upper and lower limits
of the tank concentrations were well above both the detection
limits and the NSW concentrations.
After examination of these data, questions should arise as
to their significance. In effect, what can we learn from such
data? Several trace elements are found in elevated concentrations
in aquarium water (Table 2; Figure 2). Some of these metals
have extremely high concentrations relative to NSW; tin has
already been mentioned as having concentrations over 200,000
times above normal, but Thallium, Titanium, Aluminum, Zinc,
Cobalt, Antimony, and Copper all have concentrations of over
95 times normal. Conversely, of the detected elements, relatively
few are substantially lower than normal. Although Sulfur,
Boron, Strontium, Silicon and Vanadium had lower tank concentrations
than in NSW, only Vanadium was present at less than about
50 % of normal levels.
In the remainder of this article,
I will examine the abundance patterns of the detected chemicals,
as well as some other factors, and try to determine if there
is any easily evident reason for such patterns. Furthermore,
I will try to assess the significance of such patterns and
associations.
Table 2. Average values
of Natural Sea Water and Tank Study Values Compared to
Detection Limits.
These data are in descending order with the element
found in the highest relative concentration in the tank
listed first. All values are in parts per million ( ≈ mg/kg). Blank
cells indicate that the data are not available. Values
that are “0.000000” do not indicate a value of zero, but
rather indicate the actual value is less than 1 part per
trillion (the average concentration is less than 10-12).
The variance measures in the average tank data
are the sample standard deviations. Arsenic has no variance
measure in the study as it was only found in one tank. |
Element
|
Natural Sea Water
|
Test
Detection
Limits
|
Average Tank Values
± Variance
(Mean ± Sstd)
|
Value as a Proportion of NSW Average
|
Average
|
Low
|
High
|
Average Tank
|
Detection
Limit
|
Tin
|
0.000000
|
0.000000
|
0.000001
|
0.005
|
0.095
± 0.01
|
200725
|
10531
|
Thallium
|
0.000012
|
|
|
0.01
|
0.015 ± 0.005
|
1250
|
815
|
Titanium
|
0.000010
|
0.000000
|
0.000014
|
0.001
|
0.007 ± 0.001
|
735
|
104
|
Aluminum
|
0.000270
|
0.000003
|
0.001080
|
0.01
|
0.173 ± 0.070
|
640
|
37
|
Zinc
|
0.000392
|
0.000003
|
0.000589
|
0.001
|
0.212 ± 0.021
|
540
|
2.55
|
Cobalt
|
0.000001
|
0.000001
|
0.000006
|
0.001
|
0.0002 ± 0.0001
|
154.5
|
848.9
|
Antimony
|
0.000146
|
|
|
0.01
|
0.018 ± 0.007
|
125.5
|
68.47
|
Copper
|
0.000254
|
0.000032
|
0.000381
|
0.001
|
0.024 ± 0.005
|
96.03
|
3.93
|
Nickel
|
0.000470
|
0.000117
|
0.000704
|
0.005
|
0.024 ± 0.006
|
51.11
|
10.65
|
Arsenic
|
0.001723
|
0.001124
|
0.001873
|
0.01
|
0.020
|
11.61
|
5.80
|
Iodine
|
0.050760
|
0.025380
|
0.063450
|
0.01
|
0.447 ± 0.518
|
8.80
|
0.197
|
Phosphorus
|
0.071300
|
0.003100
|
0.108500
|
0.01
|
0.328 ± 0.745
|
4.60
|
0.140
|
Lithium
|
0.172500
|
|
|
0.005
|
0.666 ± 1.462
|
3.86
|
0.029
|
Molybdenum
|
0.009590
|
0.008823
|
0.010070
|
0.005
|
0.016 ± 0.017
|
1.94
|
0.521
|
Barium
|
0.013740
|
0.004397
|
0.020610
|
0.0005
|
0.015 ± 0.008
|
1.10
|
0.036
|
Potassium
|
380
|
|
|
0.1
|
405.2 ± 61.1
|
1.07
|
0.00026
|
Magnesium
|
1272
|
|
|
0.05
|
1326 ± 138.9
|
1.04
|
0.000039
|
Sodium
|
10561
|
|
|
0.05
|
10850 ± 1246
|
1.03
|
0.000005
|
Calcium
|
400
|
|
|
0.05
|
400.4 ± 85.1
|
1.00
|
0.00013
|
Sulfur
|
884
|
|
|
0.05
|
789.6 ± 68.9
|
0.89
|
0.000057
|
Boron
|
4.60
|
|
|
0.05
|
3.935 ± 1.42
|
0.86
|
0.011
|
Strontium
|
13
|
|
|
0.0005
|
6.786 ± 1.69
|
0.52
|
0.000038
|
Silicon
|
2.810000
|
0.028100
|
5.620000
|
0.05
|
1.270 ± 1.30
|
0.45
|
0.018
|
Vanadium
|
0.001527
|
0.001018
|
0.001782
|
0.005
|
0.00002 ± 0.0000
|
0.01
|
3.27
|
Chromium
|
0.000208
|
0.000104
|
0.000260
|
0.001
|
Not Detected
|
4.81
|
Cadmium
|
0.000079
|
0.000000
|
0.000124
|
0.0005
|
Not Detected
|
6.35
|
Manganese
|
0.000027
|
0.000011
|
0.000165
|
0.0005
|
Not Detected
|
18.21
|
Yttrium
|
0.000022
|
0.000007
|
0.000027
|
0.0005
|
Not Detected
|
22.50
|
Iron
|
0.000056
|
0.000006
|
0.000140
|
0.005
|
Not Detected
|
89.61
|
Beryllium
|
0.000000
|
0.000000
|
0.000000
|
0.0005
|
Not Detected
|
2777.8
|
Silver
|
0.000003
|
0.000000
|
0.000005
|
0.01
|
Not Detected
|
3710.6
|
Lead
|
0.000002
|
0.000001
|
0.000036
|
0.01
|
Not Detected
|
4826.3
|
Mercury
|
0.000000
|
0.000000
|
0.000002
|
0.01
|
Not Detected
|
24925.2
|
 |
Figure
2. Average tank concentrations of the tested elements
as a proportion of their concentrations in NSW. Note
the vertical scale is logarithmic with each major horizontal
line being ten times the value of the one below it.
|
Materials and Methods
The data from this study are in the form
of several independent single samples. As such, the data do
not consist of replicate samples of a single treatment, and
they can not be easily tested statistically to determine the
significance of variations. However, I should point out that
such tests were never anticipated, nor planned for. Rather,
this was to be a descriptive study of several aquaria to allow
the description of "an average reef tank." Within
the purview of descriptive statistical analyses is a relatively
powerful tool: correlation analysis. This is an analytical
procedure using assumptions of a normal distribution. I am
not testing for such normality; instead I am assuming it.
All of the samples were taken from systems that were being
maintained with the explicit goal of maintaining coral reef
animal life, and most marine life has relatively low tolerance
for variation, it follows that the samples are likely either
normally distributed or close enough to normality that the
differences from normality should be minor.
Five different categories were simultaneously
compared in a single correlation analysis (Table 3). Those
items with numerical values, such as concentrations or physical
measurements were compared using those measurements. Other
measurements, such as the use of RO/DI water, or additives,
were entered into the correlation table with values of 1 (=
yes) or 0 (= no). There were a total of 44 factors, arrayed
over 25 tanks. In the analysis, each factor is compared with
every factor, including itself. The resulting matrix, with
44 factors in rows and columns, contained 1936 separate cells,
each with a comparison of the row factor with the column factor.
Of these, 44 were correlations of the factor with itself.
These values are routinely discarded. The remaining matrix,
containing 1892 values, contains two identical, but reciprocal
parts; for example, for every comparison of A with B, there
is a complimentary comparison of B with A. Consequently, there
were only 946 different correlation values to examine. Some
of these are trivial comparisons, such as when only one category
or value is found. Only one of the examined reef tanks used
filtered NSW as its water. Thus, each correlation with NSW
concerns only one datum per category, and as such has little
predictive potential. Similarly, Arsenic was detected in only
one tank, so all correlations with arsenic concern only the
one tank. Such one-factor correlations have little or no information
of value and were not considered further.
Table 3. Items used in
the correlation analyses; 44 items were considered,
resulting a grid of 1936 values.
Numerical Values or Concentrations of:
Trace Elements:
Aluminum, Antimony, Arsenic, Boron, Barium,
Calcium, Cobalt, Copper, Iodine, Lithium, Magnesium,
Molybdenum, Nickel, Phosphorus, Potassium, Sodium,
Sulfur, Silicon, Strontium, Thallium, Tin, Titanium,
Vanadium, Zinc,
Organic Materials or Nutrients:
Ammonia, Total Nitrogen, Nitrate+Nitrite,
Fat,
Tank Factors:
Tank Volume, Tank Age, Water Changes (size
and frequency), Sand Bed (presence and depth in inches),
Presence of :
Titanium Utensils (Probes, etc.), Skimmers,
Exports, Water type (RO, RO/DI, Tap, NSW), Salt Mix,
Use of:
Additives (Calcium, Iodine, Other)
|
The correlation
statistic may range from -1.000 to + 1.000. A correlation
of 1.00 means the association always occurs and is therefore
completely predictable; however, it may be either a positive
predictor or a negative one. A correlation value of 0.000
indicates no correlation or predictive value. There is no
standard approach to interpreting correlation data; it depends
on the data being examined. For the purpose of this study,
I define the correlations of 0.500 to 0.650 as weak, those
of 0.651 to 0.850 as moderate, and those of 0.851 to 1.000
as strong. Such correlations may be either positive or negative.
The correlation matrix was examined and the values within
the range of -0.499 to 0.499 were discarded and all others
were classified according to the above criteria.
It is important to remember that CORRELATIONS
CANNOT BE USED TO IMPLY CAUSATION. Correlations may be used
to infer some causal action, but such a cause needs to be
experimentally, or observationally, validated. It is all too
easy to examine correlative data and to imply that since factor
A and factor B are correlated, they must somehow be reacting
or behaving similarly due to some common cause. This is definitely
NOT true. We may infer a cause, but without other evidence,
such a causal relationship is purely speculative. As I have
no experimental data to work with, I will speculate a lot
in the discussion portion of this report.
In an attempt to assess potential utilization
of materials, I compared the average values found in these
tanks with the average values of trace metals in sea water
mixes using the data from Atkinson and Bingman (1999) study
of artificial sea water mixes.
Results
The correlation values showing associations
are shown in Table 4. Relatively few negative correlations
were found altogether, although some of them are interesting,
such as the negative association of Iodine concentration with
Calcium additions, and the negative relationship of Calcium
concentration and sand bed depth.
One of the reasons that some of the data
appear to be low may be an artifact of data manipulation.
The data were adjusted for differences in salinity by normalizing
the data as described in Shimek, 2002. If that were the case,
one would expect many of these elements with low readings
to have the same patterns of abundance. In other words they
would show correlations due to the normalization procedure.
That is apparently not the case. Sulfur and Boron do have
a slight positive association, 0.398, but it is still a weak
association at best, and probably indicates little of importance.
It, however, is the largest of the correlations concerning
the elements with low concentrations. Such weak associations
may simply reflect their similar patterns of low abundances
in some of salt mixes.
The only strong correlations were all positive
ones, and with the exception of the strong correlation between
Iodine and Phosphorus, all of them concern Cobalt, Tin, Zinc,
Titanium, and Copper. Vanadium is moderately correlated with
these metals as well, and it is evident that they form an
assemblage of metals which all show similar patterns of distribution
and variation (Table 4). Moderate and weak correlations are
numerous and some of them are quite interesting; negative
correlations are few. The only negative moderate correlation
is between Aluminum and Calcium, indicating tanks with high
Calcium concentrations tend to have low Aluminum concentrations.
Similarly, Aluminum and Strontium are also weakly and negatively
correlated with each other, and Strontium is negatively correlated
with a number of metals. The weak and moderate positive correlations
also indicate that many of the trace metals listed above are
correlated with other metals such as Nickel, Zinc, Aluminum
and some others.
The most interesting moderate correlations,
in my opinion, however, are those involving comparisons with
tank factors other than trace elements (Table 5). For example,
several metals are correlated with the presence of dissolved
fats in the water; additionally, the fat concentrations tend
to be higher in older tanks. Tanks which have been set up
longer also have high concentrations of nitrogenous compounds.
As we will see in the discussions, some of the comparisons
may say more about the aquarists than the tanks.
Table
4. The results of the correlation analyses of all tested
factors. Correlations
were regarded as weak when the correlation coefficient
was 0.500 to 0.650; moderate with values of 0.651 to
0.850, and strong when the correlation coefficient was
0.851 to 1.000. The values could be either positive
or negative. The correlation values are arrayed in
descending order.
|
Strong Positive Correlation
|
|
Strong Negative Correlation
|
|
Factors
|
Coefficient
|
Factors
|
Coefficient
|
Cobalt
with Tin |
0.993
|
None
|
|
Cobalt
with Zinc |
0.978
|
|
|
Tin
with Zinc |
0.978
|
|
|
Iodine
and Phosphorus |
0.944
|
|
|
Copper
with Tin |
0.870
|
|
|
Copper
with Zinc |
0.869
|
|
|
Titanium
with Zinc |
0.865
|
|
|
|
|
|
|
Moderate Positive Correlation
|
|
Moderate Negative Correlation
|
|
Factors
|
Coefficient
|
Factors
|
Coefficient
|
Copper
with Fat |
0.840
|
Calcium
with Aluminum |
-0.683
|
Copper
with Vanadium |
0.824
|
|
|
Cobalt
with Titanium |
0.817
|
|
|
Cobalt
with Vanadium |
0.814
|
|
|
Titanium
with Tin |
0.811
|
|
|
Nitrate/Nitrite
with Tank Age |
0.811
|
|
|
Vanadium
with Tin |
0.800
|
|
|
Nitrate/Nitrite
with Fat |
0.796
|
|
|
Copper
with Nickel |
0.789
|
|
|
Titanium
Probes with Silicon |
0.779
|
|
|
Vanadium
with Zinc |
0.776
|
|
|
Fats
with Tank Age |
0.772
|
|
|
Cobalt
with Nickel |
0.764
|
|
|
Iodine
with Nitrate/Nitrite |
0.758
|
|
|
Phosphorus
with Nitrate/Nitrite |
0.755
|
|
|
Potassium
with the Salt Mix |
0.750
|
|
|
Nickel
with Tin |
0.746
|
|
|
Nickel
with Zinc |
0.744
|
|
|
Boron
with Tank Age |
0.730
|
|
|
Nickel
with Vanadium |
0.724
|
|
|
Aluminum
with Vanadium |
0.709
|
|
|
Copper
with Nitrate/Nitrite |
0.696
|
|
|
All
Additives and Iodine Additive |
0.692
|
|
|
Copper
with Tank Age |
0.688
|
|
|
Thallium
with Vanadium |
0.681
|
|
|
Molybdenum
with the Salt Mix |
0.677
|
|
|
Copper
with Titanium |
0.666
|
|
|
Strontium
with Barium |
0.665
|
|
|
Lithium
with Molybdenum |
0.661
|
|
|
Antimony
with Tin |
0.655
|
|
|
Nickel
with Fat |
0.514
|
|
|
|
|
|
|
|
|
|
|
Weak Positive Correlation
|
|
Weak Negative Correlation
|
|
Factors
|
Coefficient
|
Factors
|
Coefficient
|
Zinc
with Fat |
0.649
|
Aluminum
with Strontium |
-0.581
|
Fat
with Tap Water |
0.646
|
Iodine
and Calcium Additions |
-0.558
|
Phosphorus
with Ammonia |
0.635
|
Calcium
with Sand Bed Depth |
-0.556
|
Silicon
with Exporting Materials |
0.631
|
Sand
Bed Depth and Calcium Additions |
-0.550
|
Antimony
with Cobalt |
0.626
|
Fat
with RO/DI Water |
-0.548
|
Vanadium with Tank Age
|
0.626
|
Strontium
with Antimony |
-0.530
|
Antimony
with Fat |
0.626
|
Strontium
with Vanadium |
-0.523
|
Cobalt
with Thallium |
0.617
|
Phosphorus
and Calcium Additions |
-0.519
|
Ammonia
with Tank Age |
0.612
|
Strontium
with Calcium |
-0.510
|
Antimony
with Zinc |
0.610
|
Potassium
with Exporting |
-0.509
|
Magnesium
with Sulfur |
0.610
|
Strontium
with Cobalt |
-0.509
|
Antimony
with Copper |
0.605
|
Strontium
with Titanium |
-0.503
|
Phosphorus
with Fat |
0.601
|
Magnesium
and Calcium Additions |
-0.502
|
Thallium
with Tin |
0.589
|
Titanium
Probes and Tank Age |
-0.501
|
Vanadium
with Fat |
0.584
|
|
|
Antimony
with Vanadium |
0.583
|
|
|
Aluminum
with Sodium |
0.582
|
|
|
Aluminum
with Cobalt |
0.575
|
|
|
Aluminum
with Tin |
0.572
|
|
|
Antimony
with Sodium |
0.571
|
|
|
Thallium
with Zinc |
0.564
|
|
|
Tin
with Fat |
0.563
|
|
|
Iodine
with Ammonia |
0.561
|
|
|
Sulfur
with Fat |
0.559
|
|
|
Exports
with Water Changes |
0.548
|
|
|
Thallium
with Nickel |
0.546
|
|
|
Boron
with Nitrate/Nitrite |
0.545
|
|
|
Antimony
with Magnesium |
0.544
|
|
|
Magnesium
with Sand Bed Depth |
0.543
|
|
|
Aluminum
with Zinc |
0.540
|
|
|
Phosphorus
with Tank Age |
0.540
|
|
|
Aluminum
with Nickel |
0.536
|
|
|
Titanium
Probes and Additives |
0.535
|
|
|
Zinc
with Tank Age |
0.534
|
|
|
Ammonia
and Total Nitrogen |
0.532
|
|
|
Magnesium
with Nickel |
0.532
|
|
|
Sodium
with Sulfur |
0.531
|
|
|
Titanium
with Thallium |
0.524
|
|
|
Antimony
with Titanium |
0.524
|
|
|
Titanium
with Vanadium |
0.518
|
|
|
Copper
with Tap Water |
0.517
|
|
|
Magnesium
with Vanadium |
0.516
|
|
|
Aluminum
with Copper |
0.514
|
|
|
Copper
with Thallium |
0.506
|
|
|
Water
Changes with Skimmers |
0.500
|
|
|
Table 5. Tank Factor Correlations
(from Table 4).
|
Metals
|
Metabolic
Factor
|
Tank
Factors
|
A.
With Dissolved Fat:
|
Copper,
= 0.840
|
Nitrate/Nitrite
= 0.796
|
Tank
Age = 0.772
|
Zinc
= 0.649;
|
Phosphorus
= 0.601
|
Tap
Water = 0.646
|
Antimony
= 0.626
|
|
RO/DI
Water = 0.548
|
Vanadium
= 0.584
|
|
|
Tin
= 0.563
|
|
|
Sulfur
= 0.559
|
|
|
Nickel
= 0.514.
|
|
|
B.
With Nitrate/Nitrite
|
Iodine
= 0.758
|
Phosphorus
= 0.755
|
Tank
Age = 0.811
|
Copper
= 0.635
|
|
|
C.
With Ammonia
|
Iodine
= 0.561
|
Phosphorus
= 0.635
|
|
D.
With Tank Age
|
Boron
= 0.730
|
Nitrate/Nitrite
= 0.811
|
Titanium Probes = -0.0.501
|
Copper
= 0.688
|
Dissolved
Fat = 0.772
|
|
Vanadium
=0.626
|
Ammonia
= 0.612
|
|
Zinc
=0.534
|
Phosphorus
= 0.540
|
|
E.
Sand Bed Depth
|
Magnesium
= 0.543
|
|
Calcium
Additions = - 0.550
|
Calcium
= -0.556
|
|
|
F.
With Titanium Grounding Probes
|
Silicon
= 0.779
|
|
Additive
= 0.535
|
|
|
Tank
Age = -0.501
|
G.
With Salt Mix
|
Molybdenum
= 0.677
|
|
|
H.
Calcium Additions
|
Magnesium
= - 0.502
|
Phosphorus
= -0.519
|
Sand
Bed Depth = -0.550
|
Iodine
= - 0.558
|
|
|
I.
With Exporting Materials
|
Silicon
= 0.631
|
|
|
Potassium
= -0.509
|
|
|
J.
With Water Changes
|
|
|
Change
Frequency = 0.733
|
|
|
Exports
= 0.548
|
|
|
Skimmers
= 0.500
|
K.
With Water Factors
|
Copper
with Tap Water = 0.517
|
Fat
and Filtered NSW = 0.507
|
|
|
|
|
|
Only 15 trace elements, Aluminum, Barium,
Chromium, Cobalt, Copper, Iron, Lead, Lithium, Manganese,
Molybdenum, Nickel, Silver, Titanium, Vanadium and Zinc, were
examined by both the Atkinson-Bingman (1999) sea water study
and this study. One sample in this study was Instant Ocean
water, made up with RO/DI , and the values for the trace metals
in that sample are quite close to the averages of the salt
waters from the Atkinson and Bingman (1999) study. All but
Zinc showed lower values in the tanks than in the mixes (Table
6) or the Instant Ocean Sample.
Table
6. Comparison of the average concentration values
for salt mixes (Atkinson and Bingman, 1999) and aquaria
from this study, including one aquarium set up with
Instant Ocean.
|
|
Instant Ocean
|
Average
± 1 Sample Standard Deviation
|
Difference of
|
Metal
|
Sample
|
Artificial Sea Water Mix
|
Aquaria
|
Tank-Mix Averages
|
Aluminum
|
6.480
|
6.885 ± 0.540
|
0.173 ± 0.070
|
-6.712
|
Barium
|
0.012
|
0.064 ± 0.037
|
0.015 ± 0.008
|
-0.049
|
Chromium
|
0.390
|
0.434 ± 0.040
|
<0.001
|
-0.433
|
Cobalt
|
0.077
|
0.090 ± 0.011
|
0.037 ± 0.003
|
-0.053
|
Copper
|
0.114
|
0.152 ± 0.026
|
0.024 ± 0.005
|
-0.128
|
Iron
|
0.013
|
0.067 ± 0.147
|
<0.005
|
-0.062
|
Lead
|
0.435
|
0.541 ± 0.070
|
<0.01
|
-0.53
|
Lithium
|
0.373
|
1.895 ± 4.237
|
0.666 ± 1.462
|
-1.229
|
Manganese
|
0.066
|
0.067 ± 0.019
|
<0.0005
|
-0.067
|
Molybdenum
|
0.173
|
0.251 ± 0.041
|
0.019 ± 0.018
|
-0.232
|
Nickel
|
0.100
|
0.114 ± 0.014
|
0.024 ± 0.006
|
-0.090
|
Silver
|
0.248
|
0.381 ± 0.074
|
<0.01
|
-0.37
|
Titanium
|
0.032
|
0.039 ± 0.007
|
0.007 ± 0.001
|
-0.032
|
Vanadium
|
0.148
|
0.168 ± 0.020
|
0.023 ± 0.005
|
-0.145
|
Zinc
|
0.033
|
0.037 ± 0.017
|
0.212 ± 0.021
|
0.175
|
|
|
|
|
|
|
Discussion
The first article of this series detailed
an average reef aquarium as described from this sample of
23 tanks (Shimek, 2002). Hidden inside the average values
discussed in that article are tendencies within various components
of the data to vary in consistent ways. These trends become
apparent with the examination of correlative data, for such
examinations allow the determination of similar patterns of
change that occur within the factors across all the samples.
In a very real sense, a correlation coefficient is a statistic
describing "trendiness." If two factors have a strong
correlation, they are consistently varying in the same manner.
Consequently, we can say that if X, Y, and Z are correlated
in our aquaria, then tanks with a high concentration of X
are likely to have high concentrations of Y and Z as well.
Knowing how things change together, and which things change
together, is the first step in really understanding what is
occurring in our tanks.
We have been able to guess, speculate,
and pontificate to our heart's content about how the various
factors that are important in the lives of our reef animals
change; for example, as tanks age or as we go from a smaller
tank to a larger one, but this study allows us for the first
time, to get some actual quantitative data to discuss. Additionally,
we now have analytical data that allow us to compare 23 tanks
in detail.
The examination of the correlations allows
us the opportunity to get a feel for the factors and processes
occurring in reef tanks. The systems in this study ranged
in size from 36 to 380 gallons, and in age from a few weeks
to about 10 years (Shimek, 2002). The use of correlative data
can allow us to examine trends across both size and age of
reef systems, as well as between users of various water types
and salts..
A few of the findings are somewhat surprising.
Across the size range of these tanks, there were no significant
correlations with tank size. This means that for the purposes
of describing a reef aquarium with regard to the factors tested
in this study, a 35 gallon tank is as good as one of 300 gallons.
Although none of these tanks were "nano" reefs,
within the size range of normal reef tanks, the systems were
all comparable, with no combination of chemicals or tested
factors peculiar to either larger or smaller tanks.
Several of the trace metals varied
in concert, particularly Cobalt, Tin, Zinc, Titanium, Copper
and Vanadium, and lower but still positive correlations with
Nickel and Aluminum are found. All of these metals are found
at concentrations far above those of natural sea water. Some
of these concentrations are almost unbelievably high. Tin
has an average concentration in our systems of over 200,000
times greater than in natural sea water. At the same time,
it must be pointed out that its average concentration is still
low; however, its natural concentration is very much lower.
The action of some of these metals in reef animals is not
known, for example, it appears quite likely that Titanium
may have no effect on reef animals one way or another. On
the other hand, some of these metals do have effects. Cobalt
is a required co-factor in all aerobic respiration as it is
part of Vitamin B12. Copper is also an essential and necessary
element for many animals' metabolism; however for many of
those same animals, and others, it is quite toxic at very
low levels just above their needed concentrations. Vanadium
is also very toxic and few marine animals can tolerate it
at all. Among those that can metabolize Vanadium are sea squirts,
or tunicates, and they use it as an antifouling agent to kill
or deter the growth of nearby organisms or organisms that
might overgrow them (Figure 3).
 |
Figure 3. Cnemidocarpa finmarkiensis,
a temperate sea squirt, each animal is about 1 inch
(2.5 cm) long. The animal in A is healthy: note the
body is shiny and devoid of animal and algal growth.
Cnemidocarpa and other tunicates secrete Vanadium
and other heavy metals through their tunic to kill over-growing
or fouling organisms. The animal in B is unhealthy and
for some reason appears unable to secrete its antifouling
chemicals. Note the overgrowth at the top of brownish
algae and at the bottom of whitish hydroids.
|
Increases
in many of these same metals are correlated with the age of
the tank. One explanation for that pattern would be that they
may build up with the passage of time. The same metals are
also correlated with the presence of fat in the aquarium water.
It is possible that such fat is related to the types of food
given to the aquarium, and that will be reported on next month.
If that is the case, the metals' concentrations may simply
be related to feeding and foods. It is also possible that
the fat in tank water comes from the organisms growing in
the system, and as older tanks often have more and larger
animals, they would produce more fats. One intriguing possibility
is that organisms in the system may secrete the toxic metals
as part of their suite of anti-predator and anti-competitor
chemicals. No matter what the cause, the build up of such
chemicals is a cause for concern.
The older tanks also have more ammonia,
nitrate/nitrite, phosphorus, iodine and copper than younger
tanks. Nitrate and nitrite are produced either from the decomposition
of excess food, which might be present in greater amounts
in older tanks, or by the processing of animal urine which
is mostly ammonia. The processed urine found in vertebrates,
mollusks, and arthropods will also contain Ammonia, Phosphorus
and Amino Acids, so it is likely the high levels of these
compounds simply reflect more living tissue in older tanks.
The higher Iodine levels in older tanks most likely reflect
an accumulation, either from feeding or from additives. Tank
Iodine concentrations average about 10 times NSW levels. This
biologically active element is most frequently found in algal
metabolites in marine ecosystems, and its high concentration
in the tanks may simply indicate either algal growth or the
addition of algal foods and additives. Iodine is also a toxic
material when found in high concentrations, and levels such
as these may be cause for some concern. Interestingly enough
tank iodine concentrations show a slight negative correlation
(-0.179) with the use of Iodine additives. The magnitude of
this coefficient implies that there is no correlation between
the use of Iodine additives and the final tank concentration
of this material. Probably much more Iodine is added in the
foods, but those data will be investigated next month. The
various forms of Iodine vary in biological activity and toxicity.
For the present, and with these data, we have no way of estimating
their various contributions, either positive or negative to
the system.
Additionally, given the logistics of the
situation, it was impossible to provide a way to reliably
filter the water samples at the time of collection. From the
aspects of organisms such as corals, particulates are as much
a part of the water environment as are dissolved materials.
These small particulates were not filtered prior to analysis,
and in all of the samples there are likely varying amounts
of particulate organic material. Such material could be responsible
for some of the correlative data between Ammonia, Phosphorus,
and Amino Acids, and Fat. Also the correlation of Fats with
the age of the tank, and some other factors relating indirectly
to tank age, may simply reflect the ability of older, more
"mature," tanks to generate more living particulate
material , either as zooplankton, phytoplankton or bacterioplankton.
I feel that the abundance of such plankton is relatively low
in most of our systems, but I certainly may be error. Filtering
out particulate material prior to the test would have, in
my opinion, removed data reflecting total abundances of several
elements and was not desirable.
Some of the correlations may tell us more
about the aquarists than the aquariums, per se. For example,
Titanium grounding probes are negatively correlated with older
tanks; this means they are likely to be found in newer tanks.
The probes are correlated with tanks that have regular additions
of additives. Consequently, it is likely that this means that
the newer tanks are maintained by aquarists that think that
grounding probes and additives are important. Also, Copper
levels are correlated with the use of tap water as a source
for mixing the salt water used in the tanks. This Copper likely
is related to Copper plumbing, which would be removed in RO/DI
water. The plumbing in our houses likely contributes to some
of the other metals concentrations as well; as other metals
may leach out of solders, or fixtures. The elevated levels
of Zinc may be indicative of brass fittings in the plumbing,
perhaps some distance upstream of the tap. As a general rule,
it may well be a good idea for aquarists who do not use RO/DI
water to consider some auxiliary means of removing Copper,
Zinc, or other metals.
There were some odd findings. Sand bed
depth was weakly, but negatively correlated with both Calcium
concentrations and Calcium additives, and positively correlated
with Magnesium concentrations, possibly indicating a certain
"sloppiness" in the efforts to maintain Calcium
by aquarists having deep sand beds.
Additionally, as a series of averages,
many of the trace element concentrations are lower than they
are in freshly made up artificial sea water. Whether this
indicates organism use, or abiotic chemical reactions is unclear.
Even though these levels are lower than in "fresh"
artificial sea water, they are still very much higher than
in natural sea water, and may still indicate a cause for concern.
These patterns are interesting and possibly
dependent on several factors. There is likely no single cause
for some of the effects, and it is just as likely that there
is no defined cause at all for some of them. In these latter
cases, the patterns would be the result of random factors
or happenstance. The high metals concentrations may be due
to build up in the tanks from foods, or from salt formulations,
or from the ill-conceived use of poorly formulated additives.
Other high concentrations, such as the fats and other metabolites,
may be due directly to in-tank metabolism or be caused from
food additions. Obviously, aquarist whim and preference may
be a major determinant of many of the factors such as iodine
and calcium concentrations. Unfortunately without experimentation,
we cannot determine causation, and such experimentation would
be expensive and time consuming.
For the moment, we are left with
some correlations to ponder. Next month, I will discuss the
composition of the various foods added to the tanks in this
study. From knowing what goes into these systems, and what
is there, I will try to estimate some of the materials flow
that must be occurring in the systems, and some of the consequences
of that flow and the feeding.
Acknowledgements:
This article benefited significantly
from reviews by Skip Attix, Eric Borneman and Randy Holmes-Farley,
and I thank them all for their efforts. Additionally, I would
again like to thank the participants and donors who made the
Tank Water Study possible: Mark Boenisch, Eric Borneman, Cliff
Carter, David Celentano, Allen Chantelois, Steven Collins,
Gregory Dawson, John Delery, Adrian Harris, Deborah Lang,
Matthew Mengerink, Steven Miller, Steven Nichols, John Link,
Jaroslaw Pillardy, Robert Schnell, Sandra Shoup, William Wiley
and Anonymous Contributors for contributing water samples.
I also thank Danmhippo@reefs.org,
Matthew Hennek, Matthew Davis, and Win Phinyawatana for providing
cash donations to support this venture. Without all of your
assistance, this project would not have been possible.
|