Introduction:
Hidden within a
single shell and slimy foot is the secret to one of the most
significant evolutionary success stories in all of the Animal
Kingdom. If one were to scan the entire array of animals and
total up the number of known species, the animal group with
the most species would be, without question, the insects.
The second most species-rich group, however, would be the
gastropods, or snails, in all of their slow, slimy glory.
The total number of snail species is not known, but estimates
range upwards of 50,000, and sometimes much higher.
Shells indistinguishable in details from modern snails appeared
during that part of the Earth's history that geologists term
the early "Paleozoic" period, well over 400 million
years ago. Although we don't know the anatomy of these animals,
we must presume that they were not too dissimilar from some
of today's snails, such as the Pleurtomariids. These ancient
snails were not particularly rare, but neither were they particularly
abundant or ecologically dominant. Tracking forward from this
ancient beginning, the snails show several changes in bodily
structure, at least as reflected in the changes in shell shapes
that appear in the fossil record. In many cases, there appear
to be modern descendents of these groups, so it is possible
to postulate what the ancient representatives were like. Some
of these ancient forms would be passingly familiar to aquarists;
they look to be the ancestors of animals such as the trochoidean
grazers, sand- or mud-dwelling ceriths or the small, rice-grain
sized rissoids found in many aquaria. Most other types, however,
would be recognizable only to malacologists, those scientists
who, like me, study mollusks.
 |
Figure 1. Perotrochus lucaya, collected
from a Bahamian coral reef at a depth of 300 m (1000
ft.), is a Pleurotomariid snail, a true "living
fossil" and a remnant of an ancient group once
dominant in the Paleozoic era. The slit in its shell
is characteristic of these animals, which are thought
to be similar to the ancestors of most other snails.
This animal was about 2.5 cm (1 inch) in diameter.
|
Sometime about the beginning of the Mesozoic era, when the
dinosaurs' dominance of the terrestrial world was not yet
even a dream of the lumbering reptiles of the time, one, or
several, gastropod lineages underwent a series of striking
changes in form that resonate down to us today. Some of these
changes would be obvious to the casual aquarium observer,
if that person could choose some ancient snails for his tank.
Other, equally important, changes would be less obvious. All
of these changes in form, however, came together to produce
animals whose shells look like modern whelks. These animals
were probably not the first predatory gastropods, but their
body's form is supremely adapted for predation upon soft-bodied
or sessile prey, and once they appeared, they explosively
radiated throughout the world's oceanic habitats.
The Earth's history is replete with advances in animals'
bodily form made to exploit hitherto unexploitable environments.
Until the late Paleozoic period, it appears that while soft-bodied
worms such as those presently found living in a deep sand
bed were abundant, they weren't very diverse. Additionally,
clams, while present, were rare. If we could see it, the ocean
bottom of that time would appear much different from the one
we see today, and the diversity of its life would be very
limited. At the end of the Permian epoch, 252 million years
ago, all of Earth's life almost died. By some estimates, 95%
percent of all animal types and 99% of all animal species
became extinct. This extinction event is so dramatic that
geologists use it to mark the end of Paleozoic period. When
life rediversified after this event, many changes occurred.
The old groups of animals didn't simply reappear but, rather,
their few survivors diversified, radiated and evolved to fill
all sorts of "vacant" ecological niches left by
the massive extinction event. At this time, animals began
to effectively exploit the soft ocean bottoms for the first
time. Diverse arrays of clams and worms, among other animals,
appeared. This process wasn't rapid or immediate; it took
several scores of millions of years, but by the beginning
of the Jurassic period, roughly 200 million years ago, the
ocean bottoms were again teeming with life and for the first
time, it appears that diverse and abundant life was found
in the soft sedimentary ocean bottoms. As the animals that
populated these regions evolved, so did their predators, and
one of the major groups of these predators was comprised of
the newly evolved whelk-like snails (Tasch, 1975).
What's a Whelk?
The whelks are predatory snails found
in several distinct, but probably closely related, taxonomic
groupings. Although it is not terribly difficult to describe
a whelk, and I will get to that below, several things must
be taken into account when trying to determine if the mystery
snail in an aquarium is, or is not, a whelk. First, color
doesn't matter. While I haven't seen a chartreuse or bright
blue whelk, I have seen just about every other color in their
shells from black to white to hot pink. Second, size doesn't
matter. The smallest adult whelks are only a few tenths of
an inch long, and the largest top out at over two feet in
length. Additionally, of course, tiny juveniles of relatively
large whelks are among some of the whelks most commonly found
in reef tanks. As a consequence of these two factors, illustrations
may not be very useful in trying to identify such animals.
(Nonetheless, here are some links to images; all of these
animals - and many, many, others - may be termed "whelks,"
1,
2,
3,
4,
5,
6,
7,
8,
9,
10.)
This latter point, the abundance of juvenile whelks in reef
aquaria, causes some further complications. Whelks are, in
general, very long-lived animals and estimates of adult ages
in the three to four decade range are fairly frequent. Such
a long life often presupposes a long juvenile period, and
that appears to be the case with many whelks; they may not
reach sexual maturity for several years. As in humans and
most other animals, they grow to their adult size while sexually
immature. Unfortunately for the person interested in a quick
and dirty identification, the definitive shell characteristics
- those characteristics used to distinguish the animal from
its nearest relatives - often don't appear until sexual maturity.
In snails, these "definitive" characters often involve
thickening of the aperture's outer lip and changes in the
aperture's shape. Consequently, while it is often relatively
easy to identify an animal as a generalized "whelk,"
particularly the small juveniles that appear in reef aquaria,
it is often very difficult to put a definitive and
specific name on it.
Figure 2. Generalized shell characteristics of
whelks (modified from Keen and Coan, 1971). Not all
whelks have all of these characters, and the characters
may occur in just about any combination. Note that the
front of the shell, as the animal is crawling on the
substrate, is at the bottom in the illustrations.
|
The generalized whelk shell form (Figure 2) is fusiform or
biconical. That is, the shell may be thought of as spindle
shaped, or as two cones joined at their wide base with the
pointed apical ends facing to the front and rear. Although
people generally hold a snail shell with one pointed end up
and the other down, the animal crawls with one of these ends,
the one ending in a trough, directed forward, and the other,
the apical, or the end with the sharp point, directed to the
rear. It is conventional to discuss the shell with the orientation
shown in Figure 2. The apex is at the top and the siphonal
canal, or notch, is at the bottom. If the animal is held in
such a manner, its aperture will generally be on the right
side when viewed with the aperture facing the observer. If
observed from the top, above the apex, the animal coils in
a clockwise direction. Such a coiling pattern resulting in
the aperture appearing on the animal's right side, is termed
"dextral." Most snails exhibit dextral coiling,
but a few, including some common whelks found along the Gulf
of Mexico's northern coast, do it the other way and coil counter-clockwise.
Such coiling is referred to as sinistral
coiling. (1,
2
- and here is a dextrally
coiled near relative of the illustrated sinistral animal).
Sinistral coiling is rare; well over 99 percent of the shelled
snails coil to the right. Why they do this is unknown.
The shell characters illustrated in Figure 2 are exceptionally
variable. They vary between species, and the various combinations
of the characters are used to distinguish species. Unfortunately,
they may also vary within a specific species. For example,
one researcher categorized over 2400 combinations of color,
lamellar height and number, and shell proportions in the common
intertidal whelk, Nucella
lamellosa, of the Pacific Northwest. Additionally,
some of the characters are labile, meaning that while they
are genetically determined, the extent of that genetic expression
may be triggered by some environmental characteristic. For
example, in the frilly dogwhelk (Nucella lamellosa)
mentioned earlier, some populations effectively lack the lamellae
that characterize the species. If individuals from one of
these populations are put into an aquarium that receives the
outflow from one of the snail-eating crabs found in the region,
Cancer
productus, some chemical given off by the crab will
induce the production of lamellae as the snail grows. Because
snails grow and add shell only around their aperture, we know
that the older parts of such animals lack lamellae and the
younger parts have them.
Figure 3. Nucella lamellosa, a whelk from
the Northeastern Pacific with highly variable shell
morphology. Notice the animal on the left is effectively
smooth, lacking the lamellae that give the species its
name. The shell on the right has the lamellae. Color
variations such as seen in these two specimens are common
in this species.
|
From the aspect of the aquarist or the person trying to identify
such animals, there is also the problem of the ambiguity of
many of the terms used to describe the shells. For example,
the difference between a varix and a lamella is really one
of degree. There is no discrete difference between a varix
and a lamella. In one sense, a varix is a low rounded lamella
and a lamella is a flattened tall varix. To make things even
more fun, some whelks are considered to have lamellae on top
of varices (the plural of "varix" is "varices").
Other terms describe shell characters that are equally "fuzzy."
Unfortunately, there is nothing we can do about this delightful
situation; practically, it means that it may be very difficult
to identify an animal from a written description. One observer's
varix is another's lamella and vice versa. For any
readers who occasionally read the comments on my online forum,
this is one reason I generally ask for images of snails. Likewise,
the variability seen in the apertural characters, and their
importance in the species descriptions, is the reason that
I ask for images that show the apertures in crisp, clear focus.
These problems notwithstanding, it is generally pretty easy
to determine if a snail is a whelk. Whelk shells are generally
biconical or broadly fusiform.
Their aperture is typically oval. The animals typically, but
not always, have an operculum
on the back of their foot which plugs the aperture when the
animal withdraws into its shell. If such an operculum is present,
it is made of protein and typically is brown, golden or black;
and it is never calcareous and round, but rather oval, crescent-shaped
or somewhat "leaf-shaped." Whelks always have a
siphonal canal with an anterior siphonal notch. The notch
is generally quite distinctive, although the canal may be
short and twisted. All other sculpturing is variable and dependent
upon at least species, and maybe upon environment.
Whelk Lookalikes
In aquaria, basically two other snail
types may be confused with whelks; these are the smaller columbellids
and the strombid conchs. Columbellids are small, seldom attaining
sizes greater than about four tenths of an inch (one centimeter).
They may appear quite whelkish, but although they often have
an anterior notch at the front of their shell, they generally
lack a true siphonal canal. Additionally, they often have
columellar folds, which are uncommon or lacking in most whelks.
Columbellids are rare in reef tanks; the only common one is
the small species sold as "Strombus maculatus"
by several vendors. Actually
a columbellid snail in the genus Euplica or Pyrene,
its basic shape and aperture without a distinct siphonal canal
indicate it is not a whelk (here
is an image of an actual Strombus maculatus). Strombids,
commonly called "conchs," are quite "whelkish"
in shell morphology; however they lack a siphonal canal, although
they often have a prominent siphonal notch. Strombids have
well-developed eyes, with visible "eyeballs" on
the ends of stalks. No whelk has an eye that looks remotely
like this. All whelk eyespots are small back dots located
at the base of their cephalic tentacles, found on each side
of their head. Whelks eat meat, thus are all predatory or
scavengers; however, no strombid can eat flesh, and because
of this strombids are perfectly good reef aquarium animals.
Figure 4. This small columbellid (Euplica
or Pyrene species) is often misidentified as
"Strombus maculatus." It is a whelk
look-alike that is a highly beneficial grazer found
in many reef aquaria. Notice that although it has a
siphonal notch and visible siphon, shown in the left
image, it really doesn't have a siphonal canal. It grows
to a length of up to 8 mm (0.3 in).
|
|
Figure 5. Some strombid whelk look-alikes. Top:
Strombus gigas, the queen conch. The proboscis
with the mouth at its tip is similar to that found in
whelks, and the siphonal notch is also present. Bottom:
Strombus alatus, the Florida fighting conch.
Pictured is the front end of a half-buried animal showing
its eye, with a visible "eyeball," which is
characteristic of the strombids. All strombids have
such eyes, while no whelk does. Thus, the appearance
of the eye, alone, can serve to distinguish the beneficial
strombids from the predatory whelks.
|
|
What Really Makes a Whelk a Whelk?
As with books, one cannot really judge
a snail by its cover or, in this case, its shell. Because
of the group's vast diversity many snails are bound to look
alike that are, at best, distantly related. The true whelks
are distinguished from other snails by the characteristic
anatomy of both their external and internal soft parts. If
the basic ancestral snail was something like the Paleozoic
Pleurotomariids discussed previously, numerous changes must
have occurred before the animal could be considered to be
whelk.
Snails are differentiated and characterized by the process
of torsion. Torsion is a 180° rotation of the animal's
visceral mass just behind its head. In essence this converts
the straight gut of the ancestral mollusk into a "U"
shaped gut, with the anus situated, in the "idealized"
form, directly above the head. In practice the anus always
is some distance off center, and generally lies to the right
of the midline, under the shell's right front edge. A snail's
body extends behind its head and then rises upward in a mass
that contains most of the internal organs or "viscera."
This "visceral hump" is what the shell covers. In
some snails, such as the limpets, this mass is a simple low
cone, and the shell is simply a calcareous conical covering
of the hump. In most snails, including the whelks, the hump
is much larger and extends upward. It is coiled, and this
causes its calcareous cover to turn into the characteristic
coiled snail shell. This shell, with its internal mass of
guts and other goodies, is relatively heavy and lies on the
animal's back as it moves along. In doing so, it creates a
space in front of the visceral mass, but under the shell and
behind the snail's head. This space is called the mantle cavity,
and it is lined by a mass of tissues referred to as the mantle.
The mantle lines the snail's aperture and secretes the shell
from some folds along its leading edge. It also continues
up under the shell and can secrete more shell material to
thicken the shell from underneath.
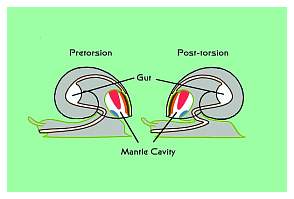 |
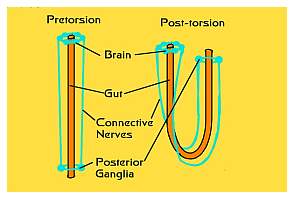 |
Figure 6. The process of torsion is THE one unique
and defining characteristic of all gastropods or snails.
No other types of mollusks undergo torsion. Left:
Torsion results in the shell and body mass being turned
180° relative to the head-foot mass. This brings
the anus from the rear to the front of the animal. Right:
The relationship of the pre- and post-torsional nerves
and gut. Torsion occurs in all snails when they are
larvae, regardless of whether or not they have shells,
and regardless of whether or not the shell, if any,
is coiled.
|
The mantle cavity in the whelks contains a number of important
organs and structures. The left front edge of the mantle extends
outward to form a fleshy tube that is often quite extensible.
This tube, called the siphon, may often extend as much as
one shell length in front of the snail and is generally held
upward at an angle to the substrate. The animal inhales breathing
water through the siphon. In some whelks, the siphon is completely
covered by shell material; in others the shell protects only
the bottom part of the fully extended tube.
Figure 7. A diagram of a generalized, and hypothetical,
whelk as seen by its prey as it approaches. The body's
and mantle cavity's structures are labeled.
|
Figure 8. Nucella lamellosa, a whelk,
dissected by one of my students, with its mantle cut
open to show some of the structures shown in Figure
7. The snail's body is to the left, out of the image.
|
As the water from the tube passes into the mantle cavity
from the left front, it passes over an organ referred to as
the osphradium. This structure is an oval or diamond-shaped
mass of pleated ridges lined with chemosensory nervous tissues.
In effect, it is the snail's equivalent of a nose or "sniffer."
Whelks often have exceptionally good abilities to smell their
prey from a distance, and when they are hunting, they move
their siphon around to find scent, similar to a bloodhound
sniffing while on the trail of its prey. This behavior may
be seen in reef aquaria by watching Nassarius snails
in search of food. Nassarius are perfectly good whelks,
differing from the typical whelks only in that they are not
predatory, but wholly scavengers. Nonetheless, they can still
follow the scent trail upstream to their food, the delicious,
juicy remains of the recently deceased, from several meters
away.
After the water has passed the osphradium, it encounters
another large mass of pleated ridges, the gill, and gas exchange
takes place. Then it passes to the right and first flows over
a glandular area that secretes a lot of mucus, called the
hypobranchial gland; then over the urinary opening or kidney
pore; next it passes by the genital structures, and finally
over the anus on the way out of the right side of the mantle
cavity.
Unlike the trochoidean grazing snails, such as Turbo
and Trochus species, the whelks have well-developed
copulatory organs. They also perform internal fertilization,
laying eggs enclosed in tough proteinaceous capsules. A few
whelk species lay eggs that hatch to produce swimming larvae.
Most whelks, however, deposit capsules that hatch to release
fully developed juvenile snails, and therein lies one major
problem for aquarists. Many of these capsules are amazingly
rugged and do a very good job of protecting the developing
snails within them. They can easily pass through the collection,
cleaning, transportation and curing processes that occur with
live rock only to hatch sometime after the rock has been put
into an aquarium. The length of time from capsule deposition
to hatching for most tropical whelks is not known, but some
of their temperate counterparts have impressively long encapsular
periods. They may take anywhere from three to 13 months from
deposition to hatching. Aquarists who neglect to carefully
examine their live rock and remove such egg capsules may acquire
"a gift that truly keeps on giving" for a long time.
Each female whelk may deposit dozens to hundreds of egg capsules,
and these may hatch over a several week period, months after
deposition. Each capsule typically releases up to a half dozen
or so voracious little whelks. These animals are predatory
from the moment they leave the capsule and some may cause
significant damage even when very small.
Figure 9. Whelk egg capsules. Left: A
Neptunea pribiloffensis female depositing her
egg mass. When she is finished, the egg capsule mass,
made of hundreds of capsules each containing thousands
of eggs, looks like a corn cob. Typically, these eggs
take 13 months to hatch from their capsules. Right:
The egg capsules of a Ceratostoma foliatum. Here
each capsule is separate and contains less than 100
eggs, which will hatch after about three months. Regardless
of the number of eggs in the capsule, generally less
than six small snails will hatch out of the egg capsules
of any given whelk. Those that survive eat the other
eggs to nourish their own growth.
|
Why Whelks are Problems in Aquaria
These animals' internal anatomy is
not particularly important except for one thing: compared
to the common grazing snails, they have very highly modified
foreguts and stomachs. The typical ancestral and primitive
mollusk's foregut, the part of the gut found between the mouth
and the stomach, contains an organ, the tongue-like radula,
which abrades and collects algal cells. Although often likened
to a rasp, the radula in these animals is more like a leaf
rake; possessing rows of relatively flexible teeth. When the
animal grazes, the radula acts like a tongue and "licks"
the substrate. During this process, the teeth dislodge and
sweep up unicellular algae such as diatoms. The flexible nature
of these animals' teeth is the major reason that most molluscan
grazers won't eat something such as hair algae: they simply
can't cut it loose from the substrate. The radula in these
grazers sweeps fine particles of food into their mouth where
they are bound in mucus, produced by salivary glands, and
conveyed to the stomach. The very complex stomach typical
of herbivorous mollusks has internal modifications to ensure
that only tiny, and precisely sized, particles of "vegetable"
material are sent out of the stomach to the midgut glands
to be digested. The stomachs of most clams, including tridacnids,
grazing snails such as the trochoideans and strombid conchs
all possess the same basic architecture. Such animals are
obligatorily herbivorous; they can be nothing but vegetarian
in their choice of food.
Whelks, on the other hand, are obligatorily carnivorous.
Their radula is no leaf rake! Instead of dozens of
modified flexible radular teeth in each row, whelks typically
have only three robust teeth with long sharpened scimitar-like
cusps in each row and, of course, the radula may contain many
hundreds of rows of teeth (here
is a beautiful scanning electron microscope image of the "business
end" of such a radula, from the whelk Murex
brandaris). The teeth are discarded as they are used
up and replacements are added as it becomes necessary. These
teeth are designed either to lacerate flesh or to first cut
through bodily coverings, such as shells, and then to lacerate
flesh. These animals' radula is very strong, and is located
at the end of a long proboscis that may extend great distances
out of the false mouth that exists at the front of their head.
The actual mouth is located on the tip of the proboscis. It
is not unusual for a whelk only an inch long to be able to
extend its proboscis a foot or more. The radula is located
near the end of the proboscis just behind the mouth, and by
extending the proboscis, the mouth can be pushed into a burrow
or tube to tear apart and eat the animal living within. As
the radula rips into the food item, salivary glands produce
lytic, or digestive, enzymes that start digesting the prey
as it is being eaten alive. The slurry of partially digested
prey tissues and bodily fluids is pumped into the stomach
and transferred directly to the midgut or digestive glands
for final digestion. As no sorting of the food is necessary,
whelks have lost the complicated primitive stomach and have
replaced it with a simple "U" shaped bend with openings
to the digestive glands. These animals cannot eat anything
but slurries of flesh and blood.
Figure 10. The lacerating radular teeth of two
Northeastern Pacific whelks. Each row of teeth contains
three teeth, one on each side and one in the center.
The radula may contain over 100 rows of teeth. Animals
in these two species are predominantly predators on
polychaete worms. The scale bars are 1 mm (Shimek, 1984).
|
One of the other properties of some whelks that makes them
such efficient predators is their ability to bore or drill
holes completely through calcareous shells such as the
plates covering barnacles and clam shells. These specific
whelks, which often carry the common name "drills,"
don't have any particular modification to their radula that
allows them to cut holes into a shell. The radular teeth are
proteinaceous; by themselves they cannot cut through the calcareous
"limestone" that comprises a snail, clam or barnacle
shell, no matter how vigorously they are rubbed over it. However,
these particular whelks have a gland in their foot that secretes
a group of chemicals that act as a chelating agent. Chelating
agents are chemicals that have an affinity for some metal,
in this case, calcium. The affinity these chemicals have is
so strong that they can literally pull the calcium out of
the calcium carbonate crystalline matrix of the clam or barnacle
shell. The shell basically turns into a pasty substance and
the radula just cleans it out of the way. The animal moves
its radula in a circular motion on the shell's surface and
periodically "wets" the area with the glandular
secretion from its foot. In very short order the shell can
be perforated. A small whelk is able to cut through a normal
clam shell in a couple of hours, and a large whelk could easily
drill through the thickest part of a Tridacna shell
over the course of one night. Once the shell has been pierced,
the whelk extends the proboscis though the hole and the radula
rips up the clam's body and ingests it.
Figure 11. Diagram of the side of a whelk showing
the proboscis and foregut structures. Top: This
shows the proboscis retracted into the cephalic hemocoel,
or blood cavity, at the animal's front end. With the
proboscis retracted, the true mouth is hidden as the
proboscis is pulled completely into the animal.
|
Figure 12. This whelk, a small Neptunea pribiloffensis,
was feeding on a group of polychaete worms living in
a hole when I removed it for measurement. The proboscis
is seen partially extended and was retracting when I
took the picture; fully extended, the proboscis was
about twice the shell's length.
|
Whelks in Reef Tanks
With the exception of one group, whelks
are not animals that are, or should be, welcome in a normal
reef aquarium. The exceptional whelks that do well in reef
aquaria, and which are good neighbors to all animals in the
reef tank, are the nassariids. These animals, mostly in the
genus Nassarius, but also including a few other small
genera, are typical whelks in all regards except their diets.
They are specialized to eat only carrion. In reef tanks, they
eat excess meaty food before it can rot, and they eat recently
deceased or dying organisms. Probably as a result of their
specialization upon carrion, which in nature is found on the
surface of sediments, nassariids typically have a proportionally
shorter proboscis than other whelks. They can't reach deep
into spaces to eat worms, nor can they drill holes through
clam shells. They can, however, and do clean up excessive
meaty foods very efficiently.
Figure 13. Nassarius vibex. Nassarius
species are some of the few reef aquarium safe whelks;
they are true scavengers and eat only either dead or
dying animals.
|
Figure 14. Some common Caribbean whelks which
may be found in reef tanks, as they are typically imported
on live rock. Left: Fasciolaria tulipa,
the tulip shell. These animals reach lengths of 20 cm
(8 inches), but small juveniles are sometimes found
in aquaria. Center: A whelk in the genus Cantharus.
These animals reach lengths of 5 cm (2 inches). They
are very commonly found in aquaria. Right: The
Horse conch, Pleuroploca gigantea. The adult
animal's body is black and is visible extending toward
the sand from this specimen's aperture. Individuals
of this species are some of the largest shelled snails;
adults may reach lengths exceeding 60 cm (2 feet). Small
juveniles are very attractive, having a bright orange
shell and red body. They are quite frequently encountered
in aquaria.
|
The more standard whelks are often long-lived animals. As
with many such animals, they would do well in a tank dedicated
to their specific needs. Additionally, these animals are often
beautiful; not only are their soft parts often brightly colored,
the shells of some of the whelks are among the most beautiful
and desirable sea shells. The whelks that most often enter
aquaria are inadvertent hitchhikers on live rock; juveniles
of some of the common Caribbean species. Still others are
purchased by unknowing aquarists assuming they are some kind
of grazing snail. In either case, their appearance in an aquarium
is "bad news." Here
is a slide show of what can happen when a whelk, in this case
an individual of Cantharus cancellarius, is placed
in a typical reef tank. If these attractive animals were not
so predatory they likely would be welcome additions to any
reef tank. Unfortunately, they are, so they are not. If they
cannot be maintained in a system of their own, whelks found
in reef tanks should be removed and humanely killed; freezing
is a good method. Put the animal in a cup of tank water and
put the cup in a freezer overnight. Then clean and examine
the shell; with some luck you will have one of the more beautiful
sea shells as a curio and reminder of the immense diversity
of coral reef animals.
Figure 15. Not all whelks eat worms, clams or
snails. These two individuals of Beringius kennicottii
were photographed eating the detached anemone (Urticina
crassicornis) shown laying on one of the snails.
The anemone had effectively been completely "cored;"
only its outer tissue layer remained. The snails are
about 10 cm (4 in) long (Shimek, 1980).
|
|