According to tradition,
the answer to the old English riddle, "Why is a duck?"
is "Because one of its legs is both the same." A
similar whiff of good, healthy surrealism is often present
in discussions of invertebrate animals in the marine reef
aquarium hobby. The majority of invertebrates are decidedly
odd and unfamiliar to most people, so perhaps the many examples
of such wackiness that occur from time to time may be excused.
However, given the familiarity that I thought most aquarists
have with fish, I have always expected discussions of fishes
to show a sounder grasp of reality. Although many aquarists
seem able to relate to and understand the practices of fish
husbandry, I have seen numerous instances in the recent past
indicating that, while the level of misunderstanding that
permeates the discussions of invertebrates may be exceptional,
there is plenty of misunderstanding about the fishes as well.
As a result, for a number of years now, I have wanted to do
a short article, or three, on the attributes of fishes simply
to discuss some of their basic features with the hope of piquing,
in some small way, further interest in them as marvelous examples
of functional morphology.
I suppose some readers may be asking themselves,
"Why should an invertebrate zoologist feel qualified
to discuss fishes?" After all, being an invertebrate
zoologist, it might seem obvious that I don't have a background
in ichthyology. Well, just as obviously, seeing much of what
is written and discussed in the hobbyist "literature,"
it is certainly apparent that having expertise in a topic
is no prerequisite to writing about the topic. Indeed, it
may be a hindrance; if someone actually has the background,
his or her ethics may prevent becoming involved in the "speculations
under the guise of fact" that seem to permeate the hobby,
particularly in some of the online forums, where common sense
is uncommon and supposition and superstition take the place
of fact and true scholarship. But I digress; I think that
all professional zoologists probably got their start studying
vertebrates. Most undergraduate university programs are organized
to teach vertebrate biology first, and then, finally, ascend
to the true and glorious path of righteous enlightenment,
the study of invertebrates. So, like most other zoologists,
I was originally trained in the ways of vertebrate animals,
and I think that training has given me, as an author, sufficient
background to cast a few lines into the pond of ichthyological
lore in the hope that I might hook a tale or two.
"If one could
conclude as to the nature of the Creator from a study of his
creation it would appear that God has a special fondness for
stars and beetles."
John B. S. Haldane
Haldane, a rather famous atheist and, probably, the pre-eminent
physiologist in the middle-third of the twentieth century,
responded with the above statement to a question about what
he could discern about God from his study of biology. One
might reckon, in addition, that He (She? It?) also had a special
fondness for fishes. If one were to pick a typical animal
from that part of the Earth covered by water, it would likely
be a fish. I don't mean to imply that fishes are the most
abundant types of animals in the seas, far from it, that distinction
probably resides with the copepods; however, fishes as a whole
are probably the most diverse group of animals living in the
water, as opposed to the benthos, of the oceans.
A Bit of Variety
Coral reef aquarists tend to oversimplify
things. We often hear statements such as, "Corals do
this," or "Fish do that," as if there were
only one species of either corals or fishes. This simplification
is, in the best case, a convenient shorthand for dealing with
a diverse group of disparate and dissimilar animals. Too often,
however, such a simplification seems to be a declaration of
ignorance that reflects a profound misunderstanding of the
animals involved and how to care for them. Nonetheless, in
the aquarium hobby, fishes probably fare better in this regard
than do most animals simply because most reef fish species
are distinctive and may often be easily distinguished from
other species. Often, probably because shallow-water fishes
are visually oriented, color patterns have developed that
are specific to individual species. After all, many advantages
accrue to fish from being able to distinguish animals of one's
own kind, not the least of which is the ability to find a
mate. We tend to take for granted that fish can see pretty
well; that is to say that they can visually assess a distant
object with some fine resolution and perhaps color. There
is no indication, however, that this was a property of the
animals that were early twigs on the bush of chordate evolution.
Quite to the contrary, the most basal animals on the chordate
lineage, the lancelets (Branchiostoma
= Amphioxus), lack eyes. The most primitive living
fishes, such as lampreys, have rudimentary eyes, while their
distant cousins, the hagfishes, lack these sense organs altogether,
although in this latter case the absence of eyes may represent
a secondary loss from an ancestor that once possessed them.
Nevertheless, good vision is a characteristic of reef fishes,
and such vision would have facilitated the development of
species-specific color patterns. As humans also have good
vision, we can distinguish the same (presumably) color pattern
signals that the fish themselves are using to discriminate
differences in gender and species. Consequently, in these
cases, the aquarist can tell a lot more about them than is
possible to easily discern from corals or many other animals.
In fact, the variety of fish types is rather bewildering;
many different types of fishes are alive today, including
several thousand species found on various coral reefs alone.
Such a diversity of form and function could make any article
discussing them as a group a futile exercise in triviality.
Notwithstanding the diversity apparent in coral reef fishes,
in terms of types of body forms, ancient fishes were probably
more diverse in general types, even though each of these types
was likely less species rich than the types found today. Recognizable
protochordates, including fossils called Haikouella
(2)
and Pikaia
(2),
were something like "super" lanclets, having been
found in the fossil record from rocks formed from sediments
deposited as long ago as the geological period referred to
as the early Cambrian epoch, roughly half a billion years
or so ago. These animals were not fishes, but they gave hints
of what was to come. Evidence of early true fishes has been
found in rocks of the Ordovician (1,
2)
period, around 400,000,000 years ago. Most of this evidence
consists of fossilized enameled teeth (only vertebrates can
make enamel), and bony scales. Whole skeletons are rare, and
many of these early fish were small, a centimeter or less
in size.
Although a lot of other different lineages of fishes have
evolved, most of these have gone extinct, and living fishes
fall into a relatively few major lineages (see, for example,
Turner, S. and Miller, R. F. 2005. New
Ideas About Old Sharks, American Scientist 93: 244-252).
Fortunately, by a process of elimination, an article's focus
may be narrowed considerably by eliminating those types of
fishes not commonly found in aquaria. The jawless fishes,
such as lampreys
and hagfishes,
certainly are uncommon in even large commercial aquaria. This
is, I suppose, a pity; these primitive fishes have some "interesting"
and odd attributes. Additionally, watching a hagfish feed
"up close and personal" is a profound and emphatic
way to impart a lasting visual definition of the word "disgusting."
Most fishes with a cartilaginous skeleton, such as sharks,
rays, and ratfishes are simply too large to be suitable for
most aquaria. Lobe-fin
fishes are almost vanishingly rare today, and, being very
large (2,
3)
as well as from deep water, are essentially impossible to
collect or maintain. Almost by default, aquarists are left
with only the classical "bony fishes" as the fishes
of choice, or phrased another way, as the primary group of
fishes that may be easily kept in the home aquarium. Bony
fishes are bilaterally symmetrical vertebrates with a few
distinctive characteristics. Roughly 30,000 species of such
fishes have been scientifically described. These species are
taxonomically arranged in about 600 families in approximately
40 orders. Characters used to distinguish the various taxonomic
groupings include mouth structure, dentition, position of
the pelvic fins, lateral line shape and position, scale characters
and the number and type of dorsal fin rays. Vertebrae, the
bones surrounding and protecting the spinal cord, define the
vertebrates, a group which includes amphibians, reptiles and
their avian and mammalian descendents as well as fishes. Given
the importance of bones to the scheme of things, I thought
it would be worthwhile to briefly discuss some of their attributes.
|
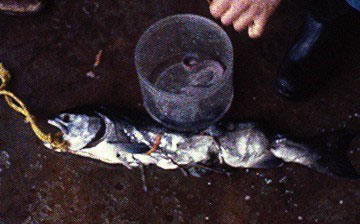 |
Figure 1. Eptatretus stouti, the Pacific
Hagfish.
Left: The large, wormlike hagfish is eating a
dead salmon suspended by a rope from the arm of a research
submersible. Hags are primitive jawless fishes that
enter their food, dead fish or marine mammals, through
all available orifices and then eat the body from the
inside out, leaving only the skin and bones. Right:
The salmon after the hags had been feeding for about
10 minutes; all the flesh was gone. A small hag is visible
in the collection bucket above the salmon.
|
Bones
Fishes, indeed all vertebrates, are
rather peculiar animals biochemically, and this is probably
related at least in part to their particular line of descent.
Recent genetic studies have shown that the chordates, including
the vertebrates, are descendent from some primitive ancestor
through a lineage that includes echinoderms. One general property
of this lineage, more so than the other major animal groups,
is the ability to metabolize and secrete calcium salts as
endoskeletal components: calcitic ossicles in echinoderms
and calcium phosphate bones in vertebrates.
In many ways, the most obvious characteristic that sets the
fishes, indeed all vertebrates, apart from all other animals
is the presence of an internal skeleton, or endoskeleton,
composed of bone. Many other animals, for example, crabs and
shrimps, have complicated exoskeletons; in those cases, however,
this skeleton is mostly proteinaceous or chitinous and, additionally,
is continuous with the exoskeleton covering their body. Although
they are mineralized, bones are unlike the calcite-containing
echinoderm ossicles, as bones are not simply crystalline material.
They are composed of a calcium-phosphate salt called hydroxyapatite
along with a significant amount of proteinaceous material.
All aquarists have seen bones and can easily visualize them.
However, I think few aquarists think about them being inside
a living animal, and particularly, they are not visualized
inside our aquarium occupants. One major feature that tends
to be overlooked is that unlike a coral's skeleton or a mollusk's
shell, vertebrate bone is a living, dynamic, tissue constantly
getting deposited, remodeled and altered in response to stress
and use. The material comprising a fish's skeleton is rapidly
and continuously metabolized and moved around, depending on
the state of the organism. From an aquarist's perspective,
it is important to consider the metabolism involving bone,
as not only does bone contain calcium, but it also contains
very large amounts of phosphate.
All animals need a lot of phosphate in their metabolic reactions,
but vertebrates need much more of it than just about any other
animals. All organisms use phosphate as part of the structural
backbone of the DNA and RNA that constitute their genetic
material. Additionally, all organisms use large amounts of
phosphate as a component of the vast number of adenosine triphosphate,
or ATP, molecules, the common "carrier" of energy
throughout living organisms. Adenosine triphosphate is a part
of just about every type of metabolic function, as it transfers
energy either to, or from, the chemical reactions that constitute
those functions. In addition to these uses of phosphate common
to all animals, vertebrates need what might be termed excessively
large amounts of phosphate because their skeleton is largely
made of phosphate salts. It often is said that one way to
cut down on the phosphate level in an aquarium is to use food
low in phosphate. This is a particularly poor argument as
it simply has the effect of starving the animals, particularly
the fish. If they can't get enough phosphate from their food
for basic metabolic processes, they will scavenge it from
their own bones, resulting in weak bones. Eventually, if phosphate
deprivation persists, the animals will die.
Functions
In addition to being phosphate reservoirs,
bones have two additional functions. They serve as protection
and they function as levers. The protective function of bones
is probably best seen in the bones of the skull. Largely fused
and immobile, these bones appear to serve two major purposes
in bony fishes: they form a protective vault around the brain,
and form points of articulation with the gill arches and the
jaw (a modified gill arch). Interestingly enough, it appears
that the bony plates that have been adapted to become part
of the skull had their evolutionary origin as bony plates
in the skin of some of the first small fishes. These fishes,
called ostracoderms, lacked jaws and were covered in enough
bony armor to make a turtle green with envy. Typically small
animals, ostracoderms were found in ancient environments where
the dominant predators appeared to be Eurypterids
or sea scorpions, relatively large arthropods related to horseshoe
crabs. It has been proposed that vertebrate dermal bone developed
in response to the predation threat such animals posed.
The axial skeleton of vertebrates probably developed in response
to natural selective pressures resulting from inefficient
locomotion. Fishes can move in a number of ways, but the one
most aquarists are probably familiar with is the use of fins
as "oars" or "sculling" devices. This
locomotion is particularly evident in fish with more-or-less
immobile bodies, such as puffers, boxfishes and seahorses.
It is much more common, though, for fish to move by rapidly
oscillating their tails. This oscillation is caused by the
contraction of muscles on opposite sides of the animal. Such
contractions tend to flex the body of a soft-bodied animal
into a series of undulations that pass down the body in a
wavelike manner. When moving in this way, forward locomotion
results from the motion of the wave passing down the body
acting against the water surrounding it. As the wave propagates
to the posterior end of the animal, the animal moves forward.
This type of locomotion is seen in eels, sea snakes and in
many types of worms. It is quite inefficient. A lot of energy
is lost simply moving the body from side to side. On the other
hand, if the body's axis is strengthened and held almost rigid
and a terminal tail fin is moved in response to these oscillations,
a very large component of the energy is spent moving the fin,
which in turn moves the animal. Such locomotion depends on
a stiff axial skeleton, which is present as the vertebral
column, along with strong lateral muscles and, often, strengthening
bony struts, such as ribs. The net result can be very rapid
locomotion, indeed; tunas, for example, can sprint to speeds
in excess of 75 km/hour (47 mph).
Figure 2. The shape and, more importantly, the skeleton
of these Barracuda
are optimized for high speed and relatively effortless swimming.
Small reef fish can't swim as fast as tuna, of course, and
chief among other reasons is that they simply don't have the
muscle mass to generate the forces necessary to propel themselves
at that speed. Even in the smallest aquaria, however, small
bursts of speed are common when fishes get startled. Among
the fastest fishes commonly kept in reef aquaria are the fusiliers,
Caesio species. These are planktivorous fishes
that are normally found swimming some distance from the reef
face nabbing small morsels brought to them on the currents.
Fusiliers share many of the structural attributes of their
high-speed cousins, and given a large enough tank, fusiliers
can be seen to move at impressive velocities.
Speed isn't everything, though. The precision movement caused
by fin locomotion alone is often a joy to watch. The delicacy
with which a Mandarin dragonet positions itself before it
strikes at a minute prey item is a testament to the advantages
of binocular vision and good eye-fin coordination. For such
locomotion to work at all, the fins must have lightweight,
but strong, internal struts. These fine bones are termed fin
rays. Bones function as levers, and when pulled by a muscle,
they transmit force to some other place; the muscles that
move a Mandarin dragonet's fins are located in the base of
the fin, yet the stiff bony fin rays transmit their energy
throughout the fins. It is well to remember, as well, that
bones do function as levers. When pulled by a muscle, they
have to transmit that force to some other place, and in doing
so they may stretch out an antagonistic muscle. Muscles, anywhere
and in anything, can only contract. Once contracted,
they will remain contracted unless they are actively re-extended.
The many small bones of a reef fish allow the fish to bend,
flex, and turn because they transmit the force of muscle contraction
through a bony lever. In doing so, they stretch out an opposing
and antagonistic muscle.
Figure 3. The lionfishes' stiff spines and fin rays
owe their functionality
to the properties of the fishes' bony endoskeleton.
Some aquarists become intimately familiar with other properties
of fins when they contact the stiffened fin rays of a Lionfish
(Pterois spp). Fitted with a potent venom apparatus,
such fins may be used either in a defensive mode or to herd
small prey into position prior to eating them. Lionfish are
not the only fish with venomous spines, of course; that property
is characteristic of the entire family of scorpion fishes.
Many other fishes also have projecting bony spines, often
at the leading edges of their fins. While not necessarily
venomous, such spines certainly can do a lot to deter predation.
Quite possibly, though, the most important attribute of
the existence of fishes, in particular, and vertebrates in
general, is so obvious that it often escapes notice: bones
continuously grow. Being internal to the tissues they have
to grow without damaging the surrounding tissues. Having a
skeleton growing inside its tissues may be vitally important
to the animal, as the bone may respond to repeated stress
by growing stronger. This means as the animal grows larger,
the skeleton not only grows larger, but it changes its shape
to match the increase in stresses put on it by the organism's
increased size. In the final outcome this means that animals
with an internal skeleton made of bones may become very much
larger than animals with an exoskeleton.
Both endo- and exo-skeletons have to change proportions as
they grow; in the final analysis skeletal functional strength
is related to the surface area where muscles and viscera are
attached as well as to the thickness of the skeletal material
that provides structural strength to the skeleton. The surface
area available for muscle attachment is measured by some factor
of the square of the skeleton's linear measurement. Skeletal
mass, however, increases as the animal's mass increases, and
this growth is in response to the cube of the linear measurement.
Additionally, muscle power is also proportional to muscle
mass, and muscle mass grows in proportion to the cube of the
linear measurement. Consequently, skeletons need to be proportionally
larger and more robust in larger animals than in smaller ones,
as the forces that the muscles can exert per unit area of
skeletal surface increase with the cube of the length, but
the skeletal surface area increases only by the square of
the length.
Figure 4. This Puget Sound King Crab, Lopholithodes
mandti, had a carapace over 60 cm (18 inches) wide
and was almost 1.3 m (4 feet) across the leg span. It
probably weighed in excess of 10 kg (22 pounds). Arthropods
larger than this are rare, due in part, at least, to
the limitations of their exoskeletons.
|
As a result, the exoskeleton of a large crab, for example,
is much thicker and more robust than is the exoskeleton of
either a smaller crab species or a smaller individual of the
same species. It has to be. The forces exerted upon it by
the muscles are much greater. However, for an animal with
an exoskeleton, increases in size can also spell trouble.
As the exoskeleton gets progressively more robust, the amount
of internal space for muscles and the viscera gets proportionally
smaller. This is probably best visualized by considering the
limb of a crab as a cylinder. In cross section, as the animal
gets bigger, if the exoskeleton is constructed of the same
material, the walls of the cylinder have to get progressively
thicker and the free volume of the cylinder gets smaller.
However, the muscles that move the limb have to lie within
the volume of the cylinder. As growth proceeds, soon there
isn't enough room in the appendage's lumen for the muscles
to move the appendage.
A crab's exoskeleton weighs about as much as the endoskeleton
of a fish of the same mass. This means that a crab the size
of a baby elephant would have to have a skeletal weight roughly
equivalent to the skeletal weight of the elephant. However,
because the elephant's muscles are not confined in all directions
by the surrounding exoskeleton, they can develop the more
extensive muscle mass necessary to move the heavier bones.
In other words, conceptually as well as actually, elephants
can "work;" large vertebrates can and do exist.
On the other hand, large arthropods on the same scale simply
can't exist. Their exoskeleton would soon be too big to move
with the muscle mass inside of it. There are a great many
ecological and evolutionary reasons that becoming large is
a "good" thing. The vertebrate body plan, based
on an internal endoskeleton made of bones, makes such growth
possible. The converse is also true, however. Such a body
plan is not a particularly good way of making a small animal.
The smallest vertebrates are reef fishes that reach an adult
size less than one centimeter long, roughly the size of a
neon tetra. Below that size, the necessary adaptations favor
an animal with an exoskeleton over one with
an endoskeleton.
Parting Thoughts
For those aquarists who are so inclined,
reef aquaria are ideal places to examine the problems of animal
form and function. The basic form of the vertebrate body plan
and the reasons for the successes inherent in this form as
it relates to larger animals are really self-evident. In terms
of the number of species, fishes constitute the most successful
vertebrates, and a large number of those are found on reefs.
Such fishes are generally larger and faster than reef invertebrates
in the area. The primary reason for this impressive radiation
of species is the presence of an endoskeleton made of bones.
Interestingly enough, the one pelagic invertebrate group that
has evolved primarily to prey upon fishes, the squids, also
contains large animals, and also is immensely successful (the
world biomass of squids is increasing due to global warming
and overfishing of larger fish that prey on small squids,
and now exceeds the biomass of humans) and, more importantly
for the subject of this article, squids also have an endoskeleton.
If you have any questions
about this article, please visit my author forum
on Reef Central.
Useful References:
Hildebrand, M. 1974. Analysis of Vertebrate Structure.
John Wiley and Sons, Inc. New York. 710 pp.
Wardle, C. S. and J. J. Videler. 1980. Fish Swimming. pp.
125-150. In: Elder, H. Y. and E. R. Trueman. eds. Aspects
of Animal Movement. Cambridge University Press. Cambridge.
ix and 1-250 pp.
|