Dinoflagellates
are single celled protists
that are superficially well-known by aquarists and laypersons
alike. First discovered by Müller in 1773, and later
described by Ehrenberg in the 1830's from examinations of
Cretaceous cherts, the dinoflagellates are thought to have
originated in the Ediacaran Era or earlier, 570 million to
one billion years ago and near the base of eukaryotic
evolution. These ancient organisms are directly or indirectly
responsible for great benefit and harm to humankind. Does
this statement sound grandiose? If so, consider that without
dinoflagellates, some 7% of the world's people would lack
food, some countries would fail to exist and many thousands
of people, now deceased, would still be alive. These tiny
cells also provide billions of dollars in economic value.
In this article, I will explain how such seemingly outlandish
claims are, in fact, true.
Dinoflagellates, composed of some 1,200 to 2,000 species
in 130 or more genera, are classified in the Phylum Dinoflagellata
and the division Pyrrophyta. Their name comes from the Greek
word dinos, meaning "whirling," with the
latter part of their name derived from the characteristic
flagella they possess. The dinoflagellates, about half of
which are photosynthetic and some of which are bioluminescent,
are important components of oceanic primary productivity.
They are found dispersed in all oceans and all ocean zones,
and can exist in pelagic or benthic zones or within host tissues.
A small percentage of them are freshwater species. They are
perhaps well-known as parasites or pathogens, and some are
even symbionts of invertebrate hosts. Dinoflagellates may
be capable of moving and swimming (all live in aquatic environments)
using two flagella. These flagella, one oriented around the
cell (the transverse flagellum), and the other oriented toward
the posterior (the longitudinal flagellum) are the diagnostic
criteria of this group.
Dinoflagellates typically have an outer covering called the
theca, or amphiesma, from which descriptive features can be
ascertained. Two broad groups of dinoflagellates can be distinguished
by the presence or absence of well-developed cellulose-containing
thecal
plates, and these groups are termed armored dinoflagellates
or unarmored (naked) dinoflagellates, respectively. The theca
may be divided into two parts by a transverse groove called
the girdle, or cingulum, that rings the cell. The part of
the theca above the girdle is the epitheca, and the part below
is the hypotheca. The girdle is the location of the transverse
flagellum. Another groove, the sulcus, runs longitudinally
and is the location for attachment of the longitudinal flagellum.
The cell's anterior end, the apex, may have an apical pore
complex in armored dinoflagellates, the function of which
is unknown. The posterior end of the cell, the antapex, often
has projecting spines or protuberances. The theca can be shed,
regenerated, or remain undeveloped during a dinoflagellate's
various life stages.
Remarkable features of dinoflagellates seem to be the rule
rather than the exception. Dinoflagellates may possess ejectile
organelles similar to the cnidarian nematocysts. Many have
numerous structures near the periphery of their cell interior
called trichocysts, features similar to those found in ciliates.
They are discharged by rapid hydration and are thought to
function in protection, toxin discharge, secretion or prey
capture. Another feature found in some species are mucocysts,
located directly below the cell membrane, which eject mucilagenous
material that may aid cells in adhering to substrates, or
may function in prey capture. A third type of ejectile organelle
is found in some species. These are the cnidocysts, and are
very similar in structure to cnidarian nematocysts. They are
less numerous than trichocysts and their function is unknown,
but they are believed to be involved in defense or prey capture.
Other unusual cellular inclusions in dinoflagellates may include
a peduncle,
pusule,
eyespots, virus-like particles, paranuclear bodies
and others, and are reviewed by Spector (1994).
Still other unusual features exist in dinoflagellates. In
almost every species, nuclear chromosomes
are permanently condensed in a condition that is similar to
what is normally seen prior to mitosis
in cell nuclei in other eukaryotes.
Furthermore, dinoflagellate nuclei contain many times the
amount of DNA found in most other eukaryotic cells, a feature
which is discussed in more depth below. At least some species
are probably polyploid.
Dinoflagellates can reproduce asexually or sexually. In the
former case, the cell divides longitudinally by fission, maintaining
a haploid
state. Each haploid daughter can, however, act as a
gamete.
The flagellated haploid cells can laterally fuse and form
a diploid
zygote
that can remain motile. Under unfavorable conditions, however,
some dinoflagellate zygotes enlarge and thicken (the hypnozygote
stage), lose motility and produce red bodies within the cell.
This diploid form, called a cyst (or dinocyst), settles to
the bottom of the water column and is a "resting stage"
in the dinoflagellate's life cycle. The cyst can have a tough
coating of a substance called sporopollenin, or it may be
embedded with calcium carbonate or silica. The cysts emerge,
or germinate, in a motile stage upon encountering favorable
environmental conditions, with another round of division,
this time by meiosis,
again resulting in a haploid cell. This type of life cycle
strategy is termed haplontic.
Some interactive and basic descriptions of mitosis and meiosis
can be found here: http://www.biologyinmotion.com/cell_division/
http://www.pbs.org/wgbh/nova/miracle/divide.html#
For more detailed information, visit:
http://www.biologie.uni-hamburg.de/b-online/e09/09b.htm
Trophic Strategies
Many dinoflagellates
are photosynthetic and are among the major primary producers
of the phytoplankton along with diatoms. This form of energy
acquisition allows some 50% of dinoflagellates to be considered
autotrophic,
although all but a few species are auxotrophic for vitamin
B12, thiamin and biotin (reviewed in
Provasoli and Carlucci, 1974). Dinoflagellates are generally
described as C3
plants, although some species may resemble C4
plants under certain conditions, and dinoflagellates,
in general, may show some characteristics of both types. The
difference between these types is whether or not three or
four carbon sugars are produced and the enzymes used to fix
CO2.
Chloroplasts are membrane bound organelles found within photosynthetic
organisms that are the primary sites of light harvesting and
photosynthesis, and contain most of the photosynthetic pigments.
The chloroplasts found in red and green algae are known to
have evolved from a symbiosis between a cyanobacterium and
a eukaryotic cell more than one billion years ago. The primary
light absorbing pigments in most plant chloroplasts are the
chlorophylls. Dinoflagellates have both chlorophyll a
(chl-a) and chlorophyll c (chl-c) whereas
most plants and green algae contain mostly clorophyll a
and, to a lesser degree chl-b. Chlorophylls d
and e also exist in algae, the former mainly in some
red algae. While some other organisms besides dinoflagellates
contain chl-c, this pigment suggests a larger evolutionary
disparity between dinoflagellates and most other "phytoplankton."
It also confers an advantage in that the photosynthetic organisms
containing multiple chlorophylls are able to effectively harvest
light energy from a broader range of wavelengths of light.
In the case of chl-b, more common in green algae, the
spectrum is shifted towards the longer wavelengths into the
green spectrum. Chl-c lacks as great a peak in the
red spectrum as chl-a, and it might be surmised that
having chl-b would be more advantageous to dinoflagellates,
since less competition for light is the primary reason to
harbor various pigments. However, it is the coupling of chlorophylls
with peridinin, a broad band light harvesting pigment, that
gives dinoflagellates a distinct advantage over other phytoplankton.
Chlorophylls are the pigments largely responsible for green
coloration in plants. The primary absorption peaks are at
430nm and 663nm, and 434nm and 666nm for chl-a and
chl-c, respectively, corresponding to the blue and
red areas of the spectrum. Because dinoflagellate chloroplasts
are unusually contained by three membranes, as opposed to
a normal one or two, it is believed that they likely have
evolved a tertiary endosymbiosis
with a plasmid
that contains the additional photosynthetic pigment complex
of peridinin
(Morden and Sherwood 2002). The orange-red peridinin pigment
absorbs very broadly, with a maximum at around 480nm and another
small shoulder at 520nm. The combined units of carotenoid-chlorophyll-protein
complexes (PCP complex) consisting mainly of peridinin, chlorophyll
a, and one of 12 to 20 proteins, form multiple complexes
where, interestingly, the interaction of chlorophyll with
the peridinin protein shifts the absorption peaks of chl-a
upwards about 10nm.
Some web images of action spectra for photosynthetic pigments
can be found here:
http://www.prozyme.com/technical/spectra/img/percp-abs.gif
http://www.chm.bris.ac.uk/motm/chlorophyll/chloroabs.gif
http://www.emc.maricopa.edu/faculty/farabee/BIOBK/pigment.gif
http://www.biology.mcgill.ca/undergrad/c441b/lect07/absorb.gif
An in-depth article about the interactions of peridinin with
chlorophyll can be found here: http://www.nat.vu.nl/bio/pdf/D03-1C6.pdf
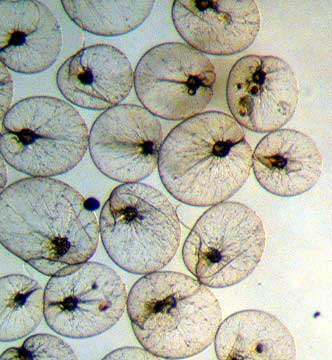 |
Here is an image of some Noctiluca, probably
N. miliaris. These are purportedly the largest
dinoflagellates. They lack chlorophyll, and eat smaller
protozoans. They are also brilliantly bioluminescent,
a fact alluded to by their name as "noctiluca"
means "night light." They are shaped rather
like a lily pad, and what appear to be "threads"
in this image are the large flagella that are used in
prey capture. Photo and caption by Ronald Shimek.
|
Other pigments are used indirectly as accessory pigments.
Some have oxidative abilities and are used in the electron
transport chains that are part of both the light and dark
reactions of photosynthesis. Some even have duplicate functions,
adding other levels of function to the photosynthetic cell.
For example, fucoxanthin
can be present as an accessory pigment in peridinin-containing
species, while in some others, it may replace peridinin. Fucoxanthin
is a common carotenoid primarily in diatoms and dinoflagellates.
Carotenoids are accessory pigments that are responsible for
predominantly yellow and orange coloration and absorb primarily
between 450nm and 550nm. Their color is usually masked by
the presence of chlorophyll, but in dinoflagellates chlorophylls
play second fiddle to peridinin. Carotenoids are composed
of carotenes and carotenols (xanthophylls). Carotenes have
numerous secondary functions, but may be most important to
zooxanthellae by acting as antioxidants. Xanthophylls consist
of oxygenated carotenes such as neoxanthin, violaxanthin and
lutein, all of which provide characteristic coloration through
absorption, either functionally or incidentally. Fucoxanthin
is a yellow-green pigment with primary absorption around 530
nm that is characteristic of dinoflagellates. Also important
in dinoflagellates are the xanthophylls dinoxanthin and diadinoxanthin,
which play roles in preventing photooxidative
damage to the photosynthetic apparatus. Also unusual for eukaryotes
is that dinoflagellates show distinct circadian rhythms, most
notably by the daily migration of the chloroplasts within
the cells.
Color: |
Name:
|
Absorption
peak (nm):
|
orange |
beta
carotene |
447,
449
|
blue-green |
*chlorophyll
a |
662,
429
|
orange-yellow |
unknown |
|
yellow |
*diadinoxanthin |
476,
446
|
orange-red |
*peridinin
(64%) |
480
|
light
green |
chlorophyll
c1, c2, c3 |
461,
583, 644
|
pink |
unknown |
464
|
yellow |
*dinoxanthin
|
470,
440
|
|
*diatoxanthin |
427,
454, 482
|
brick-red |
neo-peridinin |
|
yellow-green |
fucoxanthin |
500
|
|
zeaxanthin |
455
|
|
P-457
(neoxanthin derivative) |
457
|
*major
components
|
|
Table 1. Pigments found to be contained within
zooxanthellae of corals, comprising numerous species
of dinoflagellates.
|
Perhaps of most interest to aquarists, unarmored marine dinoflagellates
of many species are the marine symbionts known as zooxanthellae
that take up residence within the gastrodermis
of most hermatypic (reef building) coral polyps. However,
dinoflagellates have similar symbiotic roles with other marine
invertebrates including sea anemones, radiolarians,
sponges, foraminiferans,
turbellarians,
jellyfish, clams, and other groups. Much of the golden or
brown color of corals is due to the zooxanthellae, and in
particular their xanthophyll
content and composition. The degree to which this color contributes
to the corals' overall color depends on many factors, including
genetics (heritable phenotype),
pigment density, algal cell density, and production of animal-associated
fluorescing proteins. The algal cell and pigment density can
be a function of light (high light produces lower pigment/cell
density and lower light produces more darkly colored colonies
from higher dinoflagellate cell density/pigment concentration),
and nutrients (higher nitrogen causes higher dinoflagellate
cell densities). Nonetheless, symbioses with corals have enabled
coral reefs to develop, since the partnership potentially
allows the calcification rate of corals to outpace natural
degrading and eroding processes. By enhancing the success
of corals and the growth of coral reefs, dinoflagellates are
responsible for many countries' coastal buffer, the habitat
that allows for productive fisheries that provide up to 7%
of the world's protein intake, and are indirectly responsible
for billions of dollars of tourism and recreational use revenue
that centers on coral reef activities.
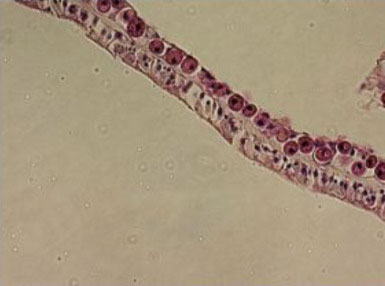 |
Small, round, naked dinoflagellates lacking flagella
are endosymbionts of corals, and are seen here as a
nearly complete monolayer in the gastrodermis of Acropora
cervicornis (upper cell layer).
Photo by Eric Borneman.
|
Not all dinoflagellates are autotrophic,
however, and some do not photosynthesize at all. They can
also exist by several variably heterotrophic strategies including
species that are phagotrophic (ingesting whole cells), saprophytic
(feeding on decaying matter), parasitic (feeding directly
on other organisms), and mutualistic (living in mutually beneficial
symbioses). Herbivory is possible in many species, and some
species have potent cellulolytic
enzymes to degrade plant cell walls. Phagotrophic species
may attach to the surface of their prey and then develop rhizopodia
that envelop the cell. Dinoflagellate species are known to
feed on eggs (particularly copepod eggs), unicellular and
filamentous algae, bacteria, and other microorganisms.
Toxic Dinoflagellates
Perhaps the most
infamous aspect of dinoflagellates is the ability of some
species to produce toxins. Blooms of toxin-producing dinoflagellates
are called "red tides" and these often-seasonal
events make news. Dinoflagellates are also responsible for
ciguatera and other shellfish poisonings. Harmful dinoflagellate
blooms produced by between 20-70 toxic species, are very similar
to blooms of non-toxic species and likely occur by competitive
exclusion. However, all toxic species are photosynthetic,
exist in estuarine or neritic (coastal water overlying the
continental shelf) areas, and produce water- or lipid-soluble
toxins.
A "red tide," more properly termed a harmful algal
bloom, is somewhat of a misnomer since the water rarely turns
red but more usually a tint of orange, resulting from the
rapid bloom and dense populations (up to 100 million cells/l)
of one or more of several species of dinoflagellates (or diatoms).
They can be problematic, for some of the rapidly blooming
species produce potent neurotoxins called saxitoxins (and
related toxins). These toxins accumulate in suspension feeders
such as edible mussels and clams, and can also accumulate
or kill fish or other animals, including man, that eat these
shellfish. The onset of symptoms, called paralytic shellfish
poisoning, can occur almost immediately upon ingestion. If
inhaled, death can occur within minutes, and while there is
no antidote, the toxin is inactivated by strong bases. Ironically,
saxitoxin and tetradotoxin in miniscule amounts is part of
the "dangerous pleasure" of eating fugu, a sashimi
of various pufferfishes. Both poisons are found and both act
in a similar fashion by blocking sodium channels. While in
Fukuoka last year, arguably the center of fugu-dining in Japan,
I opted not to roll the dice on saxitoxin/tetratodotoxin ingestion.
Other dinoflagellate species can produce brevetoxins that
cause symptoms similar to those of neurotoxic shellfish poisoning.
Massive fish kills along the Gulf Coast have been caused by
brevetoxin-producing dinoflagellate species, and aerosolization
of seaspray during red tides can cause sickness in those living
on or visiting coastlines. The most common marine toxin disease
is ciguatera, a neurologic gastrointestinal and cardiovascular
impairment caused by the accumulation of dinoflagellate-produced
ciguatoxin in contaminated fish. The higher the trophic level
of the fish, the more concentrated the ciguatoxin, and barracuda,
eels, groupers, snappers and jacks are notorious for causing
cases of ciguatera in humans after being eaten. For more information
on Ciguatera, follow this link: http://www.cdc.gov/nceh/ciguatera/default.htm
Barracuda, while tasty, are one of the fish most likely
to cause ciguatera poisoning if eaten, particularly
older and larger fish. Photo by Eric Borneman.
|
A more recent and frightening discovery
in 1988 of a toxic dinoflagellate can be found in Pfiesteria
piscicida. This species has been responsible for massive
fish kills along the Atlantic coast of the United States and
produces at least one very potent neurotoxin. The blooms,
occurring mainly in estuarine habitats, are short-lived, often
lasting only a few hours. What makes Pfiesteria so
intriguing is that there are at least 24 forms in its life
cycle, including normal non-toxic photosynthesizing forms,
toxic and non-toxic cyst forms, and a predatory form. One
toxin produced by a cyst stage stuns fish, facilitating attachment
of the dinoflagellate. The same toxin causes tissue necrosis
and open sores. Another toxin affects fish respiration. Then,
the dinoflagellate adopts a micropredatory role and feeds
on the dissolving tissue. For more information, visit: http://www.epa.gov...pfiesteria/fact.html.
Dinoflagellates in the Aquarium: "Snotty"
Dinoflagellates and Fish Parasites
Besides the precautions
that should be taken for toxic dinoflagellates which are certainly
potentially present in marine aquaria, and the obvious interest
in symbiotic species such as zooxanthellae, aquarists are
probably well-familiar with reports or experiences of a brown,
slimy, snotty algae that traps gas bubbles and covers the
surfaces of live rock, tank walls, and even corals. A web
photo of the appearance of this material can be found here.
Such periodic blooms are often reported in the spring, and
seem to correlate with deaths of fish and especially with
lethargy or paralysis and death of herbivorous snails. These
are covered extensively by Sprung and Delbeek (1994), along
with methods of eradication. Such cases may indeed be blooms
of toxic dinoflagellates. There are, however, other toxic
microalgae, other slimy snotty algae, and other algae that
trap gas bubbles. The gas bubbles are likely oxygen being
produced by photosynthesis, and of the many mat or film-forming
microorganisms, photosynthetic protists, algae, and cyanobacteria
can all appear very similar. Cyanobacteria, in particular,
are also well known for producing toxins.
During dives in both the Caribbean and
Indo-Pacific, I have noticed snotty brown material with gas
bubbles that appears to be localized areas of what are described
as dinoflagellates in reef tanks. Recently, it was found that
these accumulations on reefs are composed of chrysophytes,
specifically Chrysocystis fragilis (Schaffelke et
al. 2004). Interestingly, many chrysophytes have several
life stages, can produce toxins especially during blooms (Boenikg
and Stadler 2004), contain pigments similar to those in dinoflagellates,
and can have two flagella. They are golden brown in color,
and could be easily confused with dinoflagellates, even if
examined by microscopy. They are also correlated with tissue
mortality and bleaching signs where they cover living corals
in the wild, similar to what is seen in aquaria. For more
information on chrysophytes, visit the following page: http://www.ucmp.berkeley.edu/chromista/chrysophyta.html.
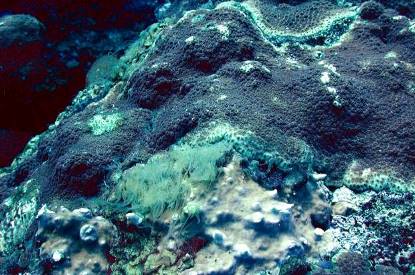 |
Chrysophytes on reef rock between Millepeora alcicornis
and Montastraea faveolata. Photo by Eric Borneman.
|
The last and perhaps best-known dinoflagellate
groups are parasites of fish, Amyloodinium ocellatum
and Cryptocaryon irritans, better known as marine velvet
and ick, respectively. These common and troublesome parasites
are covered extensively in the aquarium and scientific literature.
An article
on them has also appeared in this magazine.
Conclusion
Dinoflagellates
are an evolutionarily ancient lineage that encompasses extremely
diverse and abundant single-celled organisms. Utilizing mixotrophic
strategies and possessing numerous unusual and characteristic
features, the dinoflagellates are well-adapted for survival.
Photosynthetic species have chloroplasts
and mitochondria,
both believed to originally represent endosymbioses
with a primitive prokaryotic
cell. Dinoflagellates with a triple membrane enclosed perdinin-containing
chloroplast and a tendency to form parasitic or mutualistic
symbioses with other marine species probably represent a favorably
adaptive lifestyle. Understanding the nature of their ecology
and biology better equips aquarists to deal with both troublesome
and beneficial species. If nothing else, brown corals may
take on a whole new "light" in the eyes of their
beholders.
|