Who's On First, and What's Missing?
In the last decade
one of the most exciting, and active, areas of invertebrate
zoological research has been the study of phylogeny, or the
science of animal groups' evolutionary relationships. Biologists
have always postulated about which animal groups were related
and it has now become possible to actually determine those
relationships. Within some groups such as the vertebrates
(animals with backbones), this study has always been made
a bit easier by a good fossil record which often can reveal
useful intermediate groups. One of the best examples, the
first fossil of Archaeopteryx,
a classical un-missing "missing-link," was found
in the mid-nineteenth century. This small animal was a bit
bigger than a pigeon and, depending upon the interpretation
of the moment, was either a small dinosaur with feathers,
or a small bird with teeth, a long tail, and fingers. Dozens
of other exquisite fossils, many of them recently discovered
and described from southern China, have shown that many dinosaur
lineages had feathers. Some of these lineages obviously lead
to birds, while others had different descendents; some
of the earlier progenitors of Tyrannosaurus rex, for
example, had feathers. While the fossils of T. rex
have come from sediments that are too coarse-grained to show
either skin impressions or feathers on this delightfully cuddly
animal, it is perhaps not too far fetched to hypothesize that
adult T. rex may have retained some feathers as a signaling
device, perhaps to signal dominance or sexual prowess. Imagine
the remake of Jurassic Park showing a T. rex
with a large, erective feathered crest, as a sort of Cockatoo
from Hell. The taxonomic revision of the classification of
the vertebrates resulting from these and other fossils is
well underway, and the realization that dinosaurs and birds
are simply different expressions of the same lineage is becoming
commonplace, at least in the biological sciences.
Within the last couple of decades, researchers have made
similar strides in recognizing the relationships within groups
whose fossil record is sparse, primarily by comparing the
genetic material found in several lineages. For such determinations,
many different types of genetic material are examined and
compared. Only within the last several years have sufficient
data become available, together with the sophisticated statistical
analyses necessary to compare them. The relationships resulting
from such studies are helping to rewrite our understanding
of the evolutionary history of life.
Biologists have always been able to examine
living organisms by assessing characteristics, and have always
been able to infer degrees of "relatedness." The
problem with such assessments is that each biologist could
assess things differently- and did. This process led to the
development of several hypothesized evolutionary trees in
the animal kingdom, with little actual hard proof for most
of the branches on any of them. Often, these proposed evolutionary
pathways were based on a great degree of logic. Unfortunately,
few of these pathways were congruent, indicating that the
application of a logical methodology was rather like the application
of the concept of beauty. Logic, it seemed in this regard,
was in the eye of the beholder.
New Methods, New Answers
New, less subjective
methods of comparing animal groups started to become available
in the mid-twentieth century, primarily in response to the
problems resulting from previous subjective evaluations. These
techniques were modified, becoming more sophisticated and
quite methodologically rigorous. By the late 1980s, it was
possible to evaluate many different character sets simultaneously
and unambiguously, and from these evaluations, and by asking
the appropriate questions, to infer a series of relationships
between animal groups.
Within the last few years, then, several
different and independent methods have simultaneously been
used to evaluate the evolutionary history of the animal kingdom.
By independence, in this regard, I mean unrelated ways of
using different original data. These methods were:
1) the use of the fossil record,
2) the use of genetic material, and
3) the use of rigorous comparative techniques for examining
living animals' morphology.
These techniques have allowed the determination of relationships
to a greater level of accuracy than heretofore. One of the
more interesting outcomes is that all three methodologies
effectively resulted in the same set of relationships, and
some of these relationships were unexpected (Halanych, 2004).
This has resulted in what appears to be a paradigm shift,
similar to what took place in geology in the late 1960s when
plate tectonics supplanted earlier concepts of geological
activity.
The New Family Tree is a Shrub
One of the more interesting side-effects
of these studies has become the more-or-less formal realization
that the taxonomic hierarchy that has been in use for 250
years is seriously flawed, and in need of replacement. This
has been apparent for some time, but the ball is finally rolling
for its revision. In one of the most recent texts (Ruppert,
et al., 2003), the terms of this hierarchy, such as
phylum, class and subclass, are used only as informal descriptive
terms. The problem is that if one is dealing with a group
of interrelated organisms, animals in this case, where many
of the connections between them are now known with some certainty,
deciding how to group them becomes difficult. Basically, it
is a question of "Where does one draw the line?"
Some of the groups considered by many authorities to have
phylum status as recently as five years ago are now considered
to be part of some other group. Other groups, such as "The
Phylum Platyhelminthes," or flatworms, have been shown
to be a mistaken assemblage cobbled together from animals
belonging to several unrelated lineages. While the term "flatworm"
remains as a good descriptive term, the term Platyhelminthes
no longer applies.
The animal kingdom now appears to be comprised
of three major lineages and several smaller ones. Such lineages
are the, sometimes, diverse descendents of a common ancestral
organism and at what point the line is drawn dividing them
into separate entities is the question of the moment. The
most diverse of these lineages is called the Lophotrochozoa
and includes all of the groups sharing a common larval type
called a trochophore,
a feeding apparatus consisting of an array of ciliated tentacles.
The Lophotrochozoa consists of annelids, mollusks, and many
of the smaller animal groups. The largest lineage, in terms
of the number of species contained in it, is called the Ecdysozoa.
These are animals that must molt to grow and whose name stems
from ecdysis, the process of molting. These are the arthropods,
nematodes, and some smaller groups. The final group is a lineage
that is referred to as the Deuterostomia.
This lineage consists of the echinoderms (sea stars, sea urchins
and their kin), chordates (fish, mammals, amphibians, and
their kin) and a few smaller groups.
The animal groups within the Deuterostomia also formed a
group recognizable in earlier, more subjective schemes of
relationships. In fact, the name was first proposed in the
1880s; however, the recent re-evaluation of animal groupings
has lead to some significant shifts within it. Previously,
biologists considered that the Deuterostomes formed a more-or-less
simple linear evolutionary relationship. The echinoderms were
considered to be the basal and ancestral group, giving rise
to the hemichordates (acorn
worms and some other small bizarre polyp-like
animals), which, in turn, gave rise to the chordates, with
the tunicates being some sort of ancestral invertebrate chordate.
It now appears the basal group was some common unknown ancestor
with intermediate characters. From this ancestor came two
lines of descent, one lineage leading to hemichordates and
echinoderms, and the other to chordates, with the tunicates
as a separate offshoot of that branch. Interestingly enough,
small
fossils (and a reconstruction)
assignable to the chordates
have recently been found in some of the earliest Paleozoic
rocks, well before the time that echinoderms became common
or abundant. This would be expected if the echinoderms were
a sister group of the chordates rather than an antecedent
one.
Figure 1. The Deuterostome Animal Groups. Top:
An older conception of the relationships within the deuterostomes.
Bottom: A current conception of the relationships with
the deuterostomes. Previous relations left out the odd wormy
critter known as Xenoturbella,
as so little was known about it. Recent genetic studies place
it firmly within the deuterostomes. Modified from Halanych,
2004.
We used to think of the tunicates as a rather funky type
of chordate. The major reason for this is their specific larva
that looks a lot like a small, simplified fish. On this basis
they were considered to be pretty closely related to actual
fishes. Apparently, this is not the case. They now appear
to be considerably more distantly related. While in one sense
they are "some sort of halfway house" to chordates,
they probably would be better considered as a successful lineage
all their own, derived from a stock that gave rise, in time,
to actual chordates, but not through the tunicates.
The Tunicata
The group called
the Tunicata contains some of the oddest invertebrates. All
tunicates are marine animals and while none is found in freshwater,
a relatively large number of species may live in estuaries.
There are three subgroups of tunicates possessing some common
characteristics. As do many other invertebrate groups, they
possess a larva that undergoes a dramatic metamorphosis into
a juvenile. As mentioned above, their larvae look like small
tadpoles
or very simplified small fishes, and possess in their tail
a rod-like structure called a notochord that acts, in part,
as an antagonist for their tail's musculature. Having such
a rod in the tail allows them to swim much more efficiently
than they could without it. The larvae and the swimming ability
are transitory. Only in one class, the Larvacea, do the adults
retain the tadpole shape, and even so, as adults they do not
swim much. In the other classes, the larval tadpole shape,
the tail, and its notochord are found only in the larvae.
The notochord is one of the primary defining characteristics
of the animal group called the Chordata; in fact, that structure
gives the phylum which contains all the vertebrates, as well
as some other animals, its name.
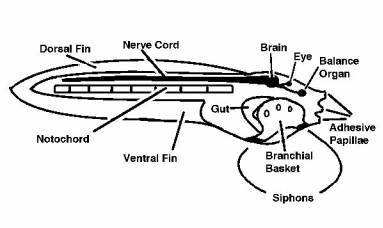 |
Figure 2. A diagram of a typical ascidiacean tadpole.
Although the gut,
branchial basket, and gill slits are present, they are not
functional and do not
open in the larva.
Showing other similarities to the chordates, all tunicates
possess openings in the front part of their gut that are considered
to be homologous with the gill slits of simple vertebrates
such as lampreys. Additionally, most adult tunicates possess
a rudimentary nervous system; as with many of their structures,
however, the larval nervous system is more sophisticated,
consisting of a main dorsal hollow nerve cord located above
the notochord with very small nerves leaving it. This is a
pattern also seen in the chordates. As their name implies,
many possess a "tunic" or outer supportive/protective
layer. This is comprised of a type of cellulose called tunicin
(Millar, 1971; Kozloff, 1990; Ruppert, Fox and Barnes, 2003).
Curiously, one of the few other places where cellulose is
found in animals is as fibers deposited within the skin.
The three subgroupings may be referred to as the taxonomic
classes of the Phylum Tunicata. Of these, only the Class Ascidiacea,
commonly called sea squirts or tunicates, is likely to be
found in aquaria. The other two classes, the Classes Larvacea
(or Appendicularia) and the Thaliacea, although almost unbelievably
common in the world's oceans, are wholly pelagic and exceptionally
difficult to maintain for any but the shortest time in aquaria.
Figure 3. A portion of a colony of a pelagic
tunicate type called a "salp." Members of
the taxonomic Class Thaliacea, salps often form chains
of connected individuals that move through the oceanic
environment using the water currents produced by the
connected individuals or zooids. Each individual here
is about 2.5 cm (1 inch) long. The body organs are located
in the colored masses; the tunics and internal filters
are visible.
|
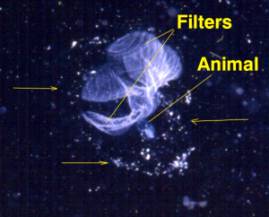 |
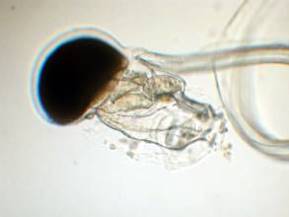 |
Figure 4. A second type of pelagic tunicate called
a Larvacean. Left: Larvaceans construct a mucous
"house" containing complex internal filters.
The small animal lives attached to the filters in the
middle of the house and eats the filters when they fill
with food. The animal (right) will then "bail
out" of the house and construct a new one from
mucus produced on its head. The tail containing the
notochord is visible coiled to the right in this image.
The edges of the house are indicated by arrows in the
figure to the left; this house was about 1 cm in diameter.
Discarded larvacean houses are one of the more common
foods of small-mouthed corals, such as Acropora.
|
There are about 1200 species of ascidiaceans. The adults
are all sessile, benthic dwelling, ciliary-mucous suspension-feeding
animals. Although there are a great many completely solitary
species, many others are gregarious, forming aggregations.
These aggregations may form from "communal settlement"
or as a result of "budding" of discrete new individuals.
Other colonies may form when many zooids share a common tunic.
All tunicates live fastened to the substrate by their cellulose
tunic.
The tunic is wholly exterior to the animal, almost completely
surrounding the body, and is secreted by the mantle, a specialized
type of secretory epidermis. The tunic is composed of numerous
chemicals, but includes tunicin, which is a type of cellulose,
as mentioned above. Only the two siphonal openings, an incurrent
and an excurrent for water passage into and out of the branchial
basket, pierce the tunic. Unlike other animals with a surrounding
cuticle, tunicates grow inside the tunic without molting.
This is probably due to their ability to resorb and redeposit
tunic materials at the mantle-tunic interface. Some species
have channels in the tunic that are continuous with internal
blood spaces, and allow for the secretion and redeposition
of tunic material as well as for the secretion of defensive
materials into the tunic.
Figure 5. A diagram of a generalized adult solitary
ascidiacean.
The tunic is shown in gray.
The two tunic openings are the incurrent, or branchial, and
excurrent, or atrial, siphons. Water enters the animal through
the incurrent siphon and passes into the highly modified throat
region called "the pharynx." The gill slits have
become highly modified and numerous, turning the pharynx into
a biological sieve called the branchial basket. The water
flows through the gill slits into another internal chamber
called the atrium. From the atrium, water exits the animal
through the excurrent or atrial siphon. The atrium also contains
the anal and reproductive openings. Gonadal products and feces
are both blown out of the atrium through the excurrent siphon
by the water currents generated by the branchial basket's
cilia.
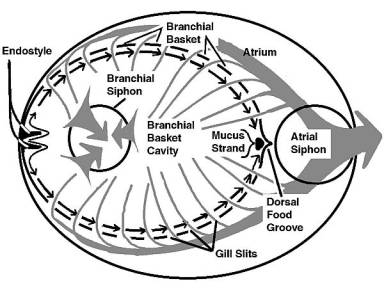 |
Figure 6. A diagrammatic representation from
above of water and mucus flow through a typical tunicate.
Water flow is shown by gray arrows, mucus movement by
black arrows. The mucus strand containing food moves
down to the mouth. The tunic and body structures are
not shown.
|
Cilia lining the edges of the gill slits pump water through
the branchial basket. In this structure, mucus is produced
from a ventral groove or gutter called the endostyle. A primary
component of this mucus is indistinguishable from vertebrate
thyroid hormone, and the endostyle is often regarded as the
thyroid gland's evolutionary precursor. The mucus flows as
a sheet from the ventral groove dorsally over the branchial
basket's inner surfaces. If you have seen living tunicates,
the terms dorsal and ventral may seem rather odd here, and
are used only to denote "morphological" directions.
The part of the branchial basket closest to the atrial siphon
is considered dorsal, and the ventral edge is on the other
side of it. The terms dorsal and ventral come from the orientation
of the larvae, and really have little meaning in the adult
due to the drastic metamorphosis that occurs between the larval
and adult stages.
Figure 7. A transparent tunicate, Clavellina
huntsmani, showing the filtering apparatus visible
through the tunic. Mucus used in the filter is produced
by the endostyle and moves up over the branchial basket
to be collected in the other pinkish linear object,
the dorsal lappets.
|
Very fine particulate organic material, mostly bacterioplankton
or small phytoplankton, is filtered from the water and is
caught on the mucous layer lining the branchial basket. Tunicates
are awesomely effective suspension-feeders; even small ones
can filter hundreds of liters of water per day and remove
well over 95% of its bacteria.
Figure 8. A shallow water tunicate-dominated area off
the British Columbia
coast. The small orange tunicates are the gregarious species,
Metandrocarpa dura.
The elongate stalked forms are the solitary species Styela
montereyensis. The smooth
gray forms, mostly in the background, are compound forms called
Distaplia smithi.
Figure 9. Left: A specimen of a tunicate, Styela
montereyensis, (see the preceding figure) dissected by
one of my students several years ago. The cut and opened tunic
is the white structure in the background. The branchial basket
has been torn, but some of the structure is still visible.
The gonads are found throughout the animal. Right:
A portion of the branchial basket of this animal photographed
through a microscope. The oval structures crossed by the horizontal
bars are the filtering gill slits.
The mucus, with its adherent food, is moved by ciliary action
to a food groove located on the dorsal midline of the branchial
basket. From there it is moved, in the food groove, into the
mouth. A very short esophagus transfers the food-laden mucus
to a bag-like stomach. Associated with the stomach is a large
structure called the digestive gland. The function of the
digestive gland is not fully understood, but it probably contributes
to digestion and absorption of food. The short, straight intestine
leaves the stomach and leads to a rectum and anus. Feces are
deposited in the atrium, and flushed out with the excurrent
water passing out the excurrent siphon.
Several species of tropical colonial sea squirts contain
symbiotic cyanobacteria growing in the branchial basket. These
cyanobacteria, in the genus Prochloron, appear to function
much like the zooxanthellae found in many other invertebrates.
The tunicates possess an open circulatory system; one that
is largely without vessels. Blood flows through large tissue
spaces or blood channels in the tissues. Arteries, veins,
and capillaries are usually absent. They have a heart, but
it is a simple tube with walls that contract to force the
blood through it. Unlike the heart in most animals, this heart
is capable of reversing its beat. Generally, the heart beats
about a hundred times in one direction, stops for a moment
and then beats about a hundred times in the other direction.
Most tunicates appear to have channels for blood flow through
the gills; these have sometimes been called blood vessels,
but they have a very simple structure. Consequently, the gill
region of the branchial basket probably functions as a respiratory
organ, but little direct evidence supports this.
In some squirts, the blood contains odd
rare-earth chemicals, commonly vanadium or niobium. These
metals were once thought to assist in respiration, but are
now known to be anti-fouling or anti-predator defenses (Stoecker,
1980; Young, 1986). Other chemicals that appear to be primarily
defensive are found in the blood. Blood serum containing these
metals and other noxious chemicals either leaks through the
epidermis, or is secreted by it and oozes through the tunic.
Additional, exceedingly acidic, defensive chemicals are found
in the tunic secretions of a few species. These secretions
have pH values of about one.
Many of the organs or organ systems present in many animals
are reduced or absent in adult tunicates. They have no specialized
excretory or osmoregulatory organs. They deposit their nitrogenous
wastes as solid crystals of guanine or uric acid in the tunic.
This is termed "storage excretion," and the animals
often look like they are dusted with a fine layer of white
dots, resulting from these granules in the tunic. The reduction
of systems is also reflected in the nervous system, which
is reduced to a small ganglion generally located between the
siphons, above the atrium. Additionally, most adult tunicates
have little capability for movement or locomotion; however,
colonies in several tropical genera including Didemnum
are capable of regular movement. These move by secreting new
tunic in the direction of locomotion and releasing old tunic
at the posterior end. The tropical genera that are mobile
all contain cyanobacteria (Birkeland, et al., 1981)
which may provide them some additional energy to use for motion.
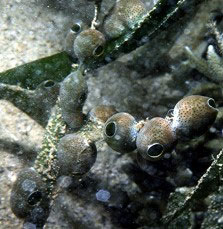 |
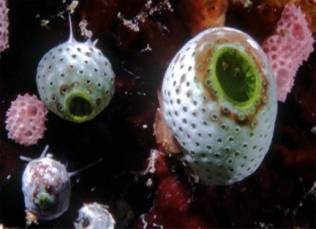 |
Figure 10. These barrel shaped colonial tunicates
are didemnids, and these images were taken in Palau.
The colonies are mobile. On the left they have
crawled up eel grass blades, on the right they
were on reef rubble. The green color is due to symbiotic
cyanobacteria.
|
Some temperate compound ascidiaceans, such as Eudiastoma
or Diplosoma species, also move by colony expansion.
The colony grows rapidly and may send out large blob-like
processes over the bottom. Connections between these processes
and other parts of the colony may break and the smaller fragments
may then grow new extensions, and the process is repeated.
While any single part of the colony remains in place, the
colony moves by extensions that move out over the substrate.
Reproduction
All tunicates are
hermaphroditic, and are often self-fertile. The gonads can
develop just about anywhere in the animal. The gonoducts run
parallel to the intestine and empty into the atrium near the
anus. Gametes released from the gonoducts develop into non-feeding
"tadpole" larvae.
These larvae look very much like tiny tadpoles.
They have a small, globular body with a long tail. The tail
contains a supportive gelatinous rod, the notochord, composed
of large cells. There are muscles on either side of the tail
and the notochord. The notochord acts as an internal hydrostatic
skeleton for the antagonism of muscle flexion. These small
larvae swim rather well. A fin is typically found running
completely around the length of the tail along its midline.
The dorsal, hollow nerve cord runs above the notochord from
a hollow, anterior brain to the tip of the tail. There are
typically a single eye, or photoreceptor, and a statocyst,
or balance organ, associated with the brain. The gut is present
but as the larva is non-feeding both the mouth and the anus
are sealed. Generally, these larvae have only a few, non-functional
gill slits. Several adhesive papillae are found on the animal's
front end.
The larvae exit the parent's atrial siphon and swim for a
short period before selecting a substrate, often by making
exceptionally precise choices (Svane and Young, 1989; Young,
1989; Stoner, 1994). During this period they are often conspicuous
and might appear to be prime food for planktivorous fishes.
This does not appear to be the case, however, and it appears
that some of the larvae are also protected by toxic chemicals
(Lindquist, et al., 1992; Lindquist and Hay, 1996).
The larvae glue themselves, nose first, to the substrate using
the secretions of anterior adhesive papillae. This is followed
by an exceptionally rapid, drastic and complex metamorphosis.
Epidermal cells covering the animal's surface contract and
within a period of 10 to 15 seconds they crush the tail and
its inner notochord, muscles, brain, nerve cord, and sensory
structures into a mass of tissue debris. The rest of the viscera
rotate about 90 degrees by differential growth, and the mouth
and anus open. The dorsal ganglion develops from nervous system
remnants. Little juvenile squirts start to feed a few days
after settlement. Growth can be very rapid in small animals,
with adult size reached in a few weeks. Others live longer
and grow more slowly (Cloney, 1982).
The largest solitary animals, reaching sizes in excess 30
cm in height, may live for several years. Most solitary species
are much smaller, and are probably more ephemeral. Colonial
tunicates grow by budding new individuals off the initial
individual. There is almost no polymorphism, and the small
individuals that make up the colony live inside a common tunic.
These colonies can be huge; I have seen some in the North
Pacific that have been 20 m long and 3 to 4 m wide, and they
can grow very rapidly. On occasion, I have peeled back the
edge of such a colony and found living sea cucumbers and scallops
entombed under it.
Figure 11. Overgrowth of other animals by colonial
tunicates. Left: A colonial tunicate, Aplidium
sp., that has partially overgrown and surrounded a live
sea cucumber, Eupentacta sp. Right: The
leading edge of a large mass of colonial tunicates,
possibly Diplosoma, that is in the process of
overgrowing some tube worms. The whole tunicate covered
several square meters.
|
Figure 12. Tunicate wars. The gray Diplosoma
sp. is overgrowing
the orange Ritterella sp. Overgrowth kills the loser.
Conclusion:
Tunicates are often
strikingly beautiful animals, but they have been difficult
to maintain in many aquaria for the same reason that many
other suspension-feeding animals are. As with many of these
filter feeders, tunicates are primarily bacteriovores, and
in general, our systems simply don't contain bacteria or fine
particulate material sufficient to support their growth.
Figure 12. A small gregarious tropical tunicate, probably
in the genus Clavelina.
If the conditions for normal growth are
met, there are no reasons the animals should not reproduce
in aquaria. Asexual reproduction of the colonial forms is
common in nature and should occur in aquaria. Sexual reproduction
may occur as well, particularly in the colonial forms. The
short larval lives, often only a few minutes, of some of these
animals will facilitate their reproduction in our systems.
If conditions are acceptable for the larvae, they will settle
and metamorphose into juveniles. The recently settled juveniles
are very vulnerable to predation, and may not survive in a
tank with many grazers or foraging hermit crabs. Once past
the smaller juvenile stages, however, these animals are generally
well protected from predation by their chemical and physical
defenses (Stoecker, 1980; Young, 1986) and will survive well
in aquaria.
Tunicates, like corals, will be kept for
the aesthetic value of the colony or individual. They generally
don't move, and as adults they have almost nothing that could
be called behavior; about all they can do is open and close
their siphons. Nonetheless, for the hobbyist who has a "green
thumb," tunicates can provide much colorful beauty to
a tank. In purchasing tunicates, it is important to choose
small individuals, and generally individuals that are attached
to rocks or some other substrate. In my experience, large
individuals do not seem to survive the collection and transport
process well, and additionally, when they feed they often
need far more nutrition than is found in a normal tank. Although
loose individuals can be fastened to rocks with epoxy or other
glues, the collection process often damages them when they
are removed from their substrate.
For more information on these animals,
literally dozens of web pages are devoted to tunicates, mostly
to photographs of them; run searches using: ascidian, tunicate,
or urochordata. Additionally, a lot of good up-to-date technical
information can be gathered from the issues of the Ascidian
News.
|