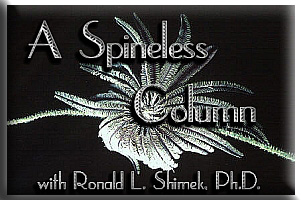
Survivors
|
Musings
A common statement
seen in print and heard at marine aquarium conferences is
something like, "Corals and other reef organisms are
delicate and are consequently hard to keep." On th surface,
this seems to be a reasonable statement. After all, reef aquarists
invest a lot of money and time to provide what they consider
appropriate conditions for these "delicate" animals.
Corals, in particular, and coral reef animals, in general,
do seem to be difficult for some hobbyists to care
for. Of course, the difficulty in caring for them is compounded
by the fact that many hobbyists seem to have their own preconceived
ideas of what the conditions are on coral reefs and these
preconceptions seem to intersect only rarely with reality.
Some of these preconceptions result from misinformation that
has made its way into the popular aquarium literature. As
in other aspects of human endeavor, in the reef aquarium hobby,
bad ideas seem to have an allure that transcends reason. Possibly
the worst of these ideas are the myths regarding temperature
and salinity. Never mind that these basic physical conditions
of coral reefs have been well-known since Charles Darwin's
pioneering publication describing the method of coral reef
formation (Darwin, 1842). Reef aquarists might find it interesting
that Darwin published his first major work essentially as
a geologist. In that book he correctly hypothesized the mode
of coral reef formation, although the collection of the final
data substantiating his theory had to wait until after the
preparations for the thermonuclear tests on Pacific coral
atolls. By the way, should an aquarist want a copy of this
book for his or her reef library, facsimile editions are available
at many booksellers. On the other hand, if the urge is for
an original, a first edition copy inscribed in Darwin's hand,
one recently became
available at auction. It could be had provided, of course,
the collector could meet the estimated starting bid of £20,000.
The longevity of this knowledge about reefs
notwithstanding, many sources still recommend keeping coral
reef aquarium temperatures in the mid-seventy degree Fahrenheit
range. This is a range that most reef animals would seldom,
if ever, see in their natural world. While some higher latitude
reefs are found in areas where these temperatures occur, and
though upwellings can cool reef waters periodically, the number
of coral reef species that can thrive at such temperatures
is relatively small, and in just about every group that could
be examined, the number of species that are found in such
regions is very low and limited (see, for example, the data
on corals in Veron, 1986, 2000). What should be obvious to
one and all seems to escape a lot of people; that is that
animals in captivity do best under the same natural environmental
conditions where their wild populations are doing the best
(Clausen and Roth, 1975; Coles and Jokiel, 1977, 1978; Highsmith,
1979a, b; Highsmith, et al., 1983; Prosser and Heath,
1991; Sorokin, 1991; Carricat-Ganivet, 2004). Research on
over 1000 reefs (summarized by Kleypas, et al.,
1999) has confirmed that the average reef temperature is 81.7°F,
and that is the temperature at which the average coral will
do best. Keeping animals adapted for this average temperature
at lower temperatures results in a drop in metabolic rate
of about five percent for each degree Fahrenheit below the
optimum temperature (Prosser and Heath, 1991). In other words,
animals whose optimum is about 82°F are "running"
at only about 75% "efficiency" at 77°F (Prosser
and Heath, 1991). Such a reduction in metabolism reduces all
physiological functions such as immunity, growth, and repair
of injury. On the other hand, corals and coral reef animals
sometimes exist very near the top of their thermal tolerance
range as well. They really push life to the limits, so that
temperature increases as minor as a few degrees above the
normal natural temperatures for their habitats can cause problems.
For animals from cold water reefs, this upper lethal limit
may be as low as 86°F, while for most reef animals it
is in the range of about 90°F.
|
Figure 1. Corals, such as this temperate cup
coral, Balanophyllia elegans, are simple animals
having only a few types of tissues and no organs. They
lack the ability to adjust to swings in their physical
environmental conditions, and must be kept near their
optimal conditions for long-term survival. For this
particular coral, that means a temperature around 50°F.
|
Similar observations can be made about
the salinity of the medium in which these creatures are kept.
Diverse coral reefs are found in areas where the water has
the salinity of non-diluted oceanic water (Sverdrup, et
al, 1942; Veron, 1986; 2000; Longhurst, 1997). The effect
of lowered salinity on both specific animals and ecological
assemblages has been known for a long time (for some examples,
see: Gosner, 1971; Zajac and Whitlach 1982a, b; Whitlatch
and Zajac, 1985; Kato, 1987; Hoegh-Guldberg and Smith. 1989;
Bulger, et al., 1990; Kozloff, 1990; Moberg, et
al., 1997; Ferrier-Pagés, et al., 1999;
Sakami, 2000; Alutoin, et al., 2001; Ruppert et
al., 2003). In fact, the deleterious effects of lowered
salinity are so well known that fluctuations in natural environmental
conditions can be tracked by the reduction in growth and well-being
of corals as recorded in their skeletal deposition (Hailey,
et al., 1994; Guzman, H. M. and A. W. Tudhope, 1998).
In spite of the preponderance of data showing the harmful
effects of lowered salinity, some authors, and many manufacturers
of artificial salt water mixes, still persist in stating in
their instructions to mix artificial sea water to abnormally
low levels; levels that will result in high stress for many
coral reef animals (see, for example: Kirschner, 1991; but
other examples in the same text, Prosser, 1991, are also informative).
The average salinity of more-or-less normal
coral reefs ranges from 34 ppt to 38 ppt, with lower extremes
being areas near large river mouths and the higher extremes
being areas such as the Red Sea where extreme evaporation
and subtidal hydrothermal activity tend to elevate the value
(Kleypas et al., 1999). Incidentally, since about 1980,
oceanographers have "officially" defined the salinity
of seawater as a dimensionless unit, referred to as S, PS,
PSU or PSS for various combinations of the words "Practical
Salinity Units" or "Practical Salinity Scale."
Salinity is now defined as the ratio of the seawater's conductivity
to that of a specified potassium chloride solution, rather
than as an actual measure of how much salt it contains. Most
biologists and many biological oceanographers, however, have,
in large part, ignored this change in nomenclature. Thus,
the recent marine biological and oceanographic literature
may express salinity either as S, PS, PSS; or, by traditionalists,
as ppt (Pilson, 1998). For aquarists who depend on hydrometers
for measuring their aquarium water's salinity, adequate salinity
for their organisms is represented by specific gravity values
in the range of 1.025 to 1.026. In spite of this, some manufacturers
still recommend mixing artificial sea water to a specific
gravity of about 1.022. Such a value results in salinities
between 28 and 30 ppt, well outside the range for long-term
survival of most coral reef organisms.
Coral reef animals are, indeed, delicate and hard to keep
alive. Anybody can prove this by getting some of these animals
and keeping them at temperatures that are abnormally low,
abnormally high, or under salinity conditions that stress
the animals to their maximum limits. Under such conditions,
is it any surprise that the animals die when the slightest
other factor goes wrong? It should not be, and neither should
the observation that many of these animals also will simply
just die from prolonged exposure to those conditions.
Survivors
Humans can live
in all sorts of environments. We generally do this by altering
our environment, by using clothing or buildings to alter our
immediate microhabitats to contain the temperature range that
our ancestors required during their evolution. Additionally,
we are mammals, and mammals often can keep their metabolism
constant over relatively wide ranges of external temperature
variation. Most animals can't do this, of course, and humans
often tend to regard these "limited" animals, such
as corals, as "delicate." On the other hand, if
corals and other coral reef animals are kept under conditions
that approximate their natural and normal environment, not
only are these animals not delicate, they are among the most
resilient and hardy of all living things.
Delicate animals, indeed! These are animals whose life spans
are so great that if they were handled competently, they could,
in many cases, outlive their keepers. Relatively few animals
can withstand the pounding of waves from hurricanes, but these
are conditions periodically seen in virtually all reef habitats.
And most of their animals survive them; indeed many acroporid
corals may require such conditions for successful reproduction
(Lafferty et al, 2004). Additionally, the shallowest
of coral reefs are subjected to extremes of both infrared
and ultraviolet radiation. Even with all of these risks to
contend with, living corals that are absolutely ancient, over
3,000 years old, have been commonly found. Some other coral
reef animals, such as sponges and many echinoderms, also know
no defined life span. Unlike the corals, they can't be easily
aged, but there is no a priori reason that they should
not get as old, or older, than the corals. Sea urchins can
exceed 200 years of age (Ebert and Southen, 2003), and sea
stars probably get every bit as old. Many of the worms, such
as the palola worms that reproduce by budding off swarmers,
but whose body stays hidden in the rocks, should be able to
live decades, at least, and perhaps longer. In temperate areas,
many snails can live well over 50 years, and some clams have
been aged at close to 200 years old. There is no reason to
suggest that coral reef organisms shouldn't be as long lived
as these.
Surviving the Worst of Times
There is another,
altogether more profound, reason to regard all these animals
as survivors, though. All animals have an evolutionary history
that dates back to a common ancestor. Changes in the genetic
materials found in all animals suggest that life that was
recognizably "animal" probably appeared on Earth
around 1,000 million years ago. Definitely small, and probably
wormy, such animals may have looked similar to either acoel
worms or the planula larvae found as early life stages in
many cnidarians such as corals.
The fossil record of animals unmistakably recognizable as
Cnidarians first appeared in the early Cambrian period about
500 million years ago. Although they didn't immediately proliferate,
reefs of one sort or another were present by the middle of
the Paleozoic Era. These reefs were formed by corals, but
they were corals that may have been decidedly unlike modern
corals in structure. Two different types of stony corals developed
in the Paleozoic, the rugose
and tabulate corals. As in modern scleractinian corals, the
coral polyp sat in a skeletal cup, or corallite. In the modern
or scleractinian corals, the corallites were subdivided internally
by septal ridges, radially formed calcareous plates. Similar
patterns are seen in the fossils derived from these ancient
animals. However, the patterns of ridges seen in all of these
types of corals are very different from each other.
Fossils attributable to tabulate
corals were first found in rocks of the Ordovician age,
but in some regards they are difficult to characterize fully.
Moore, et al. (1952) state, "The tabulates are
a group of unknown origin or exact zoological relationships,
which may well include ancestors zoantherian and alcyonarian
coelenterates." In other words, this group may be the
group that gave rise to both stony and soft corals. Their
skeletons were typically tubular and had table-like internal
platforms or "floors" called tabulae.
There were a lot of variations on these themes, and this group
proliferated in the middle of the Paleozoic era, from the
Lower Ordovician age. In a lot of regards, many tabulate coral
skeletons (= fossils) look like the skeleton of the pipe organ
coral, Tubipora musica.
Tabulate corals built reefs throughout the Ordovician, and
are found in what now covers a geographical region from Alaska,
to Baffin Island, and Texas. These particular types of corals
declined after the Ordovician and while locally present, never
seemed particularly dominant after that (Tasch, 1975). Even
if they were reduced in dominance, however, they were a major
reef-building component on reefs that were dominated by rugose
corals as well as some massive sponges called stromatoporids.
Rugose corals looked a bit more like modern corals. The skeletons
of some looked rather like a cow's
horn with septa coming from the side. Presumably a more-or-less
"normal" looking coral animal lived in the cavity.
The rugose corals were similar to the tabulate corals, in
showing an amazing amount of derivation and evolution of forms.
In some cases, the forms have been tracked through several
thousand feet of rocks deposited by sediments. Carruthers
(1910) followed the evolution of one genus, Zaphrentites
(1)
through 4000 feet of Scottish rocks showing the change from
the oldest species, Zaphrentites delanouei, through
several intermediates to the youngest representative in those
rocks, Zaphrentites disjuncta.
By the middle
to late Paleozoic era, huge
reefs were being formed. These were dominated by rugose
and tabulate corals, and by thousands of species of associated
animals. The scale of these reefs can be visualized by considering
that the fossil remnants of such reefs constitute whole mountain
ranges in Texas and elsewhere in the southwestern United States.
Then it All Changed
Over a very short
geological time frame, possibly as short as a single really
bad afternoon, but more likely over a period of about 10,000
to 30,000 years (Bowring, et al., 2001), animal life
on Earth almost ceased. It has been estimated that up to 95%
of all species in the seas went extinct. Life on land was
hit similarly hard. Whole rich lineages became extinct or
were severely reduced in numbers, including for example, ancestral
mammals. As a typical example, all the sea urchins alive today
are believed to be descendent from one genus, Miocidaris,
which survived the extinction event. Similar reductions occurred
in almost every group that survived the event (Benton, 2003).
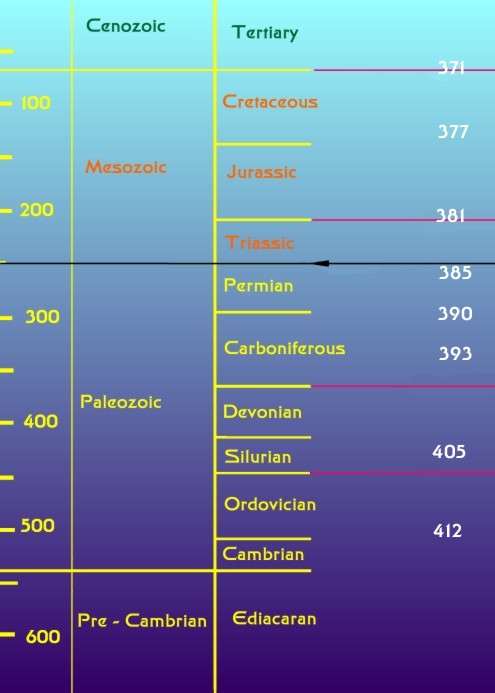 |
Figure 2. The geological time scale (modified from
Benton, 2003). The numbers on the left indicate the age in
millions of years before the present. The geological eras
are indicated in the middle column; fossil animal life became
abundant only at the beginning of the Paleozoic Era. Geological
periods, subdivisions of the eras are seen in the right column.
The periods are defined largely on the basis of the fossils
found in them, so they often are bounded by an extinction
event. The five major extinction events of the last 600 million
years are indicated by lines from the right side of the graph.
The black line indicates the Permian
-Triassic extinction event discussed in this column. The uppermost
extinction event line corresponds to the asteroid impact that
caused the extinction of dinosaurs. The numbers down the right
side of the graph indicate the length of the year in days
as determined by the microscopic study of fossil coral skeletons
(Well, 1963). The Earth's rotational period has changed dramatically
over the planet's history.
The cause or causes of this extinction event, the worst in
our planet's history, are unknown. The two leading contenders
are an impact
event similar to the one that eliminated the Dinosaurs
160,000,000 years later, and massive
volcanic eruptions in an area of present day Siberia which
could have liberated huge amounts of toxic gases into the
atmosphere; still other causes also are being considered.
Or, frankly, it could have been a combination of several different
types of these catastrophic events occurring in close proximity,
as there is some fairly good evidence for each. Whatever caused
this extinction event had a profound effect on the environment;
these effects were profound enough that they are still evident
after a quarter of a billion years. It appears that there
was a period of global warming, possibly caused by the massive
eruptions producing the flood of basalt deposits called the
Siberian
traps. These eruptions were the largest volcanic eruptions
on land in the last 600 million years, producing about 2,000,000
km3 (480,000 mi3)
of basalt (Benton, 2003); this is roughly an area 2,000 miles
long, by 1,000 miles wide covered half a mile deep in lava!
Such eruptions, and there have been several much smaller ones
within human history, produce significant amounts of what
we can call "greenhouse" gases. The greenhouse effect
of these eruptions was coupled with a period of reduced oxygen
in the atmosphere (Grice, et al., 2005; Ward, et
al., 2005). The atmospheric oxygen level was estimated
to have dropped to about 16% and that oceans became anoxic
below about 400 feet. Even in shallow waters, the oxygen tension
of the water was likely quite low. These are decidedly NOT
good conditions for life and whole groups of organisms perished.
Among them were the rugose corals, and the tabulate corals
were severely affected as well. Coral reefs disappeared. For
over 10 million years after this extinction event, at the
end of the Permian era, few coral fossils are found and no
reefs existed.
Corals recognizable as modern scleractinian corals began
to appear in the middle Triassic period. They proliferated
widely and rapidly in the Mesozoic. At the time of the dinosaurs'
extinction, the corals were about as diverse as they are today.
Several groups went extinct with the dinosaurs, but others
soon replaced them. However, the real survivors of this story
are their ancestors; those hardy animals that survived the
crash in life at the Permian period. During and for several
score million years after this extinction event, the world's
ocean was not the relatively benign place we see today. It
was a harsh, demanding environment, and these hardy animals
made it through.
Conclusion:
Marine animals that
survived that horrible period of extinctions gave rise to
all the animals we see in the seas today. The ruggedness of
those ancestral animals lives on in modern reef animals, the
corals and all others. Give them half a chance in an aquarium,
and they will do well. All they need is a warm, nicely illuminated
little sea to call their own, with some food to eat, and they
will be hardier than most aquarists can imagine.
We are now likely in the midst of another great extinction
event. It began on land with the extinction of most of the
large mammals between 10,000 and 100,000 years ago (Beck,
1996; Ward, 1997), and has continued in the seas. Recently,
it has been demonstrated that literally thousands of species
have been eliminated from the North Atlantic in the last few
thousand years by human overfishing (Jackson, 2001). Additionally,
we are well into the process of raising the world's temperature
to record high levels, and to suspect that this will lead
to a global disaster such as occurred at the end of the Paleozoic
is reasonable. Rational estimates are that over 100 species
a day are now going extinct (Benton, 2003), so the planet's
biota at the end of this century will be very different from
when it began on 1 January, 2001. These changes will particularly
impact coral reefs. Although many corals will likely persist
(Hughes, et al., 2003), it is highly unlikely that
anything familiar to one of us as a coral reef will be alive
at the end of this century (Pandolfi, et al., 2003).
It will be interesting to see how long the hardy survivors
of the coral reef can withstand this new and present peril.
Figure 3. An image of a past assemblage which is unlikely
to be seen again. These Diadema antillarum were photographed
in the Caribbean in 1981. In
1983, a disease killed most of these sea urchins.
Through their grazing activities, these urchins controlled
the structure of the reef. Although a few survived the event,
most were killed and the Caribbean reefs, in general, changed
drastically. Subsequent to this event, the dominant Acropora
species in the region have also been extirpated. The present
reefs are very different places from the reefs of 1981 and
it is unlikely that the assemblages of organisms that constituted
those earlier reefs will ever return.
Humanity is, of course, the cause of the
present extinction event. This makes it all the more important
that we treat our aquarium animals with the care and concern
that they need. By keeping our animals in their optimal physical
conditions we can at least ameliorate a small portion of the
human degradation of the reef environment.
|
If you have any questions about this article, please visit my author
forum on Reef Central.
|
References:
Alutoin, S., J. Boberg, M. Nyström and M. Tedengren.
2001. Effects of the multiple stressors copper and reduced
salinity on the metabolism of the hermatypic coral Porites
lutea. Marine Environmental Research. 52:289-299.
Beck (1996) On discerning the cause of late Pleistocene megafaunal
extinctions. Paleobiology 22(1): 91-103.
Benton, M. J. 2003. When Life Nearly Died: The Greatest
Mass Extinction Of All Time. Thames & Hudson, Ltd.
London. 336 pp.
Bowring, S. A, D. H. Erwin, Y. G. Jin, M. W. Martin, K. Davidek,
and M. Wang. 2001. U/Pb Zircon geochronology and tempo of
the End-Permian Mass Extinction. Science 280: 1039-1045.
Brandon, D. E. 1973. Waters of the Great Barrier Reef Province.
pp. 187-232. In: Jones, O. A. and R. Endean (eds).
Biology and Geology of Coral Reefs. I Geology. New
York, Academic Press.
Bulger, A. J., B. P. Hayden, M. E. Monaco, D. M. Nelson and
M. G. McCormick-Ray. 1990. A proposed estuarine classification:
analysis of species salinity ranges. ELMR Report Number 5.
Strategic Assessment Branch, NOS/NOAA. Rockville, MD. 28pp.
Carruthers, R. G. 1910. On the evolution of Zaphrentis
delanouei in Lower Carboniferous times. Geological Society
of London Quarterly Journal. 66:523-538.
Carricart-Ganivet, J. P. 2004. Sea Surface temperature and
the growth of the West Atlantic reef-building coral Montastrea
annularis. Journal of Experimental Marine Biology and
Ecology. 302: 249-260.
Clausen, C. D. and A. A. Roth. 1975. Effect of temperature
and temperature adaptation on calcification rate in the hermatypic
coral Pocillopora damicornis. Marine Biology. 33:93?100.
Coles, S. L. and P. L. Jokiel. 1977. Effects of temperature
on photosynthesis and respiration in hermatypic corals. Marine
Biology. 43:209?216.
Coles, S. L. and P. L. Jokiel. 1978. Synergistic effects
of temperature, salinity and light on the hermatypic coral
Montipora verrucosa. Marine Biology. 49:187?195.
Darwin, Charles, The Structure and Distribution of Coral
Reefs. Being the First Part of the Geology of the Voyage of
the 'Beagle.' London, Smith, Elder & Co., 1842. Also:
Darwin, Charles, Geological observations on Coral Reefs,
Volcanic Islands, and on South America: being the Geology
of the Voyage of the Beagle, under the Command of Capt. FitzRoy,
during the Years 1832-36. London, Smith, Elder & Co.,
1842-6].
Ebert, T. A. and J. A. Southon. 2003. Red sea urchins (Strongylocentrotus
franciscanus) can live over 100 years: confirmation with
A-bomb 14carbon. Fishery Bulletin.
101:915-922.
Ferrier-Pagés, C., J. P. Gattuso and J. Jaubert. 1999.
Effect of small variations in salinity on the rates of photosynthesis
and respiration of the zooxanthellate coral Stylophora
pistillata. Marine Ecology Progress Series. 181:309-314.
Gosner, K. L. 1971. Guide to identification of marine and
estuarine invertebrates. Wiley-Interscience. New York, NY.
693pp.
Grice, K., C. Cao, G. D. Love, M. E. Bottcher, R. J. Twitchett,
E. Grosjean, R. E. Summons, S. C. Turgeon, W. Dunning, and
Y. Jin. 2005. Zone Euxinia During the Permian-Triassic Superanoxic
Event. Science Online, 20 January, 2005.
http://www.sciencemag.org/cgi/content/abstract/1104323v1
Guzman, H. M. and A. W. Tudhope. 1998. Seasonal variation
in skeletal extension rate and stable isotopic (13C/12C and
18O/16O) composition in response to several environmental
variables in the Caribbean reef coral Siderastrea siderea.
Marine Ecology Progress Series. 166:109-118.
Halley, R. B., P. K. Swart, R. E. Dodge and J. H. Hudson.
1994. Decade-scale trend in sea water salinity revealed through
δ18O analysis of Montastraea annularis annual growth
bands. Bulletin of Marine Science. 54:670-678.
Highsmith, R. C. 1979a. Corals, The Inside Story. Ph. D.
Dissertation. Department of Zoology, The University of Washington,
Seattle. 322pp.
Highsmith, R. C. 1979b. Coral growth rates and environmental
control of density banding. Journal of Experimental Marine
Biology and Ecology. 37:105-125.
Highsmith, R. C., R. L. Lueptow and S. C. Schonberg. 1983.
Growth and bioerosion of three massive corals on the Belize
barrier reef. Marine Ecology Progress Series. 13:261-271,
illustr.
Hoegh-Guldberg, O. and G. J. Smith. 1989. The effect of sudden
changes in temperature, light and salinity on the population
density and export of zooxanthellae from the reef corals Stylophora
pistillata Esper and Seriatopora hystrix Dana.
Journal of Experimental Marine Biology and Ecology. 129:279-303.
Hughes, T. P., A. H. Baird, D. R. Bellwood, M. Card, S. R.
Connolly, C. Folke, R. Grosberg, O. Hoegh-Guldberg, J. B.
C. Jackson, J. Kleypas, J. M. Lough, P. Marshall, M. Nyström,
S. R. Palumbi, J. M. Pandolfi, B. Rosen, and J. Roughgarden.
Climate Change, Human Impacts, and the Resilience of Coral
Reefs. Science, Vol 301: 929-933.
Jackson, J. B. C., M. X. Kirby, W. H. Berger, K. A. Bjorndal,
L. W. Botsford, B. J. Bourque, R. H. Bradbury, R. Cooke, J.
Erlandson, J. A. Estes, T. P. Hughes, S. Kidwell, C. B. Lange,
H. S. Lenihan, J. M. Pandolfi, C. H. Peterson, R. S. Steneck,
M. J. Tegner, and R. R. Warner. 2001. Historical overfishing
and the recent collapse of coastal ecosystems. Science,
293:629-638.
Kato, M. 1987. Mucus-sheet formation and discoloration in
the reef-building coral, Porites cylindrica: effects
of altered salinity and temperature. Galaxea. 6:1-16.
Kirschner, L. B. 1991. Water and ions. In: Prosser,
C. L. Ed. Environmental and Metabolic Animal Physiology.
Wiley-Liss, Inc. New York. pp. 13-109.
Kleypas, J. A., J. W. McManus, and L. A. B. Menez. 1999.
Environmental Limits to Coral Reef Development: Where Do We
Draw The Line. American Zoologist. 39:146- 159.
Kozloff, E. N. 1990. Invertebrates. Saunders College
Publishing. Philadelphia. 866 pp.
Lafferty, K. D., J. W. Porter, and S. E. Ford. 2004. Are
Diseases Increasing in the Ocean. Annual Review of Ecology,
Evolution, and Systematics. 35:31-54.
Lessios, H. A. 1988. Mass Mortality of Diadema antillarum
in the Caribbean: What Have We Learned. Annual Review of Ecology
and Systematics. 19: 371-393.
Longhurst, A. R. 1998. Ecological Geography of the Sea. Academic
Press. San Diego. 398 pp.
Moore, R. C., C. G. Lalicker and A. G. Fisher, 1952. Invertebrate
Fossils. McGraw-Hill Book Company, Inc. New York. 766p.
Moberg, F., M. Nystrom, N. Kautsky, M. Tedengren and P. Jarayabhand.
1997. Effects of reduced salinity on the rates of photosynthesis
and respiration in the hermatypic corals Porites lutea
and Pocillopora damicornis. Marine Ecology Progress
Series. 157:53-59.
Pandolfi, J. M., R. H. Bradbury, E. Sala, T. P. Hughes, K.
A. Bjorndal, R. G. Cooke, D. McArdle, L. McClenachan, M. J.
H. Newman, G. Paredes, R. R. Warner, J. B. C. Jackson. 2003.
Global trajectories of the long-term decline of coral reef
ecosystems. Science 301:955-958.
Pilson, M. E. Q. 1998. An Introduction to the Chemistry
of the Sea. Prentice-Hall, Inc. Upper Saddle River, NJ.
431 pp.
Prosser, C. L. Ed. Environmental and Metabolic Animal
Physiology. Wiley-Liss, Inc. New York. 578 pp.
Prosser, C, L. and J. E. Heath. 1991. Temperature. In:
Prosser, C. L. Ed. Environmental and Metabolic Animal Physiology.
Wiley-Liss, Inc. New York. pp. 109-167.
Ruppert, E. E, R. S. Fox, and R. D. Barnes. 2003. Invertebrate
Zoology, A Functional Evolutionary Approach. 7th
Ed. Brooks/Cole-Thomson Learning. Belmont, CA. xvii
+963 pp.+ I1-I26pp.
Sakami, T. 2000. Effects of temperature, irradiance, salinity
and inorganic nitrogen concentration on coral zooxanthellae
in culture. Fisheries Science (Tokyo). 66:1006-1013.
Sorokin, Y. I. 1991. Parameters of productivity and metabolism
of coral reef ecosystems off central Vietnam. Estuarine, Coastal
and Shelf Science. 33:259-280.
Sverdrup, H. U., M. W. Johnson, and R. H. Fleming. 1942.
The oceans, their physics, chemistry, and general biology.
New York, Prentice?Hall, Inc., 1087 pp.
Tasch, P. 1975. Paleobiology of the Invertebrates. Data
Retrieval from the Fossil Record. John Wiley and Sons,
Inc., New York. 946 pp.
Veron, J. E. N. 1986. Corals of Australia and the Indo-Pacific.
University of Hawaii Press. 644pp.
Veron, J. E. N. 2000. Corals of the World, Vol.1-3.
Australian Institute of Marine Sciences. Townsville. 1382
pp.
Ward, P. D. 1997. The Call Of Distant Mammoths: Why The
Ice Age Mammals Disappeared. Copernicus - Springer Verlag.
New York. 241 pp.
Ward, P. D, J. Botha, R. Buick, M. O. De Kock, D. H. Erwin,
G. Garrison, J. Kirschvink, and R. Smith. 2005. Abrupt and
Gradual Extinction Among Late Permian Land Vertebrates in
the Karoo Basin, South Africa. Science Online, 20 January,
2005. http://www.sciencemag.org/cgi/content/abstract/1107068v1
Wells, J. W. 1963. Coral growth and geochrometry. Nature.
197: 948-950.
Whitlatch, R. B. and R. N. Zajac. 1985. Biotic interactions
among estuarine infaunal opportunistic species. Marine Ecology
Progress Series. 21:299-311.
Zajac, R. N. and R. B. Whitlach. 1982a. Responses of estuarine
infauna to disturbance. I. Spatial and temporal variation
of initial recolonization. Marine Ecology Progress Series.
10:1-14.
Zajac, R. N. and R. B. Whitlach. 1982b. Responses of estuarine
infauna to disturbance. II. Spatial and temporal variation
of succession. Marine Ecology Progress Series. 10:15-27.
|
|
|