Echinoderm Redux
There
are certain things we take for granted when we think of animals.
Last month, I discussed echinoderms being very strange animals
because they have, among other things, adapted a secondarily
derived radial symmetry. As soon as they metamorphose from
a larva into a juvenile animal, they become radially symmetrical
after abandoning the bilateral symmetry found in most animals.
We are all familiar with the saying, "Beauty is only
skin deep." Perhaps my perceived supposition about echinoderms'
oddity is based upon only superficial appearances which have
little to do with the actual natural history or properties
of the animal. Perhaps, if they are examined in detail, they
will be seen to be very much like all other animals internally
or in their behavior and other life history attributes.
Not a snowball's chance! If anything, these
animals are weirder on the inside than they are on the outside
(Hyman, 1955; Kozloff, 1990, Ruppert et al., 2003).
When we think of normal animals, such as worms, fishes, insects,
snails or crustaceans, they all share a certain array of preconceived
properties. The concept of "animalness" seems to
be a conservative one as far as most people are concerned.
When a group of animals such as the sponges or, for that matter,
sea anemones or corals, deviates significantly from those
preconceptions, questions about their being "true"
animals may arise. This has resulted in names such as "flower
animals" or "sea anemones," names that indicate
a basic confusion about the type of organism that is so named.
Throughout history questions have arisen as to the type of
life that such atypical organisms represent. It wasn't until
the late 1700's, for example, that sponges were accepted as
animals, and that confirmation had to await the result of
microscopic examination of their cellular structure.
This basic confusion about what it took
to be considered an animal resulted from a rather loose "gut
feeling" of what characteristics were necessary to separate
animals from all other living things. Although it might seem
easy enough to do this, deciding on what constitutes "animalness"
based on the organism's general appearance is a very difficult
proposition. This is simply due to the fact that there are
a lot of animals, and a lot of them are odd and unfamiliar.
This plethora of oddity has resulted in a rather simple "lowest
common denominator" type of definition of what constitutes
an animal. Although this definition has changed a bit through
the years, it is rather basic and straight forward. As we
now conceive of them, animals are considered to be multicellular
organisms whose cells lack walls made of cellulose or chitin
and which are incapable of photosynthesis. Such a definition
is purposefully broad, but it works pretty well to inclusively
define the animal kingdom. This definition is one that has
been created and designed as much to exclude certain organisms,
such as slime molds and various of the life forms that used
to be called protozoans, as it was to include others. Interestingly,
it also takes microscopic observation of the organism's cells
to confirm the designation of "animal." Thus, the
unaided eye alone can't unambiguously show that any given
"strange" organism is an animal. Similar and equally
specific definitions have been made for plants, fungi, and
various other groups.
Nonetheless, while such definitions work
pretty well for biologists, they really cannot be, and are
not, used by most "normal" people. Most folks look
at an organism, and if it moves and eats, it is considered
to be an animal. The conservative and preconceived definition
of an animal as being "a mobile and moving organism"
works pretty well for most of the animals we see around us.
It works passably well for most echinoderms as well. However,
they still lack many of the characteristics that people associate
with animals.
They Really Are Strange
Using
some other characteristics as yardsticks also leads to the
conclusion that echinoderms are not the normal type of animals.
As an example, people will often characterize animals as "warm
blooded" or "cold blooded." The underlying
assumption is, of course, that they have blood. Or in other
words, they have a circulatory system with a heart that pumps
fluid we can call "blood." This arrangement can
take many different forms. For example, there are animals
with one heart, such as insects, and there are other animals
with many hearts, such as earthworms. There are even animals
that have a good circulatory system which lack hearts altogether,
such as the ribbon worms or nemerteans. There are animals
such as squids or mammals whose circulatory systems keep the
blood completely enclosed within vessels. In contrast, there
are animals, such as copepods, whose circulatory systems have
no vessels at all; their blood simply bathes the cells and
tissues. There are animals with circulatory systems using
iron-based respiratory pigments, there are animals whose circulatory
systems have copper-based respiratory pigments, and there
are animals whose circulatory systems have no respiratory
pigments at all. And then there are the echinoderms who appear
to lack a circulatory system altogether.
This factor alone separates echinoderms
from most other animals. They are unique amongst larger animals
in lacking any specific system that appears to have the function
of a circulatory system. In some, structures appear to have
been derived from circulatory systems, such as the so-called
"hemal strand" in sea urchins. Unfortunately, this
strand doesn't circulate anything. But it is red and may contain
hemoglobin
In some sea cucumbers quite an elaborate
system of vessels surrounds the gut and connects it to the
body wall. This could be a good circulatory system, except
for one minor detail: nobody has been able to demonstrate
that anything circulates in it. Digested food may be transferred
to the body wall though it, but even that is doubtful, and
the system has no true and defined circulatory flow.
And Now We Can See Where We're Headed, But Only
If We Have A Head
Animals
have to eat. All definitions of what an animal is agree on
that point. Although a few animals such as corals and their
kin, and flatworms, have a gut with only one opening, the
presence of a gut generally means that animals have a gut
tube with a mouth at the front and an anus at the rear. To
have a mouth at the front and anus at the rear, animals must
have both a front and a rear. As I discussed last month,
most echinoderms lack a front end or a rear end. A front end
is defined for most animals as the end bearing the head and
mouth. In most echinoderms, the mouth opens in the middle
of one surface of the body. That surface may face down toward
the substrate, as in sea stars or sea urchins, or may face
upward toward the overlying water, as in the crinoids. In
any case the mouth is found only on an "end" of
the animal in the holothuroids or sea cucumbers.
Figure 1. Crinoids, such as the Cenometra bellis
shown here, have both their mouth and anus on the same surface.
Figure 2. Except crinoids, all living echinoderms,
such as the Diadema sea urchin shown here, have a downward
facing mouth located in the center of the oral disk.
Generally, when animals have a mouth at
one end, it is found in a structure called the "head"
containing the brain and major sensory structures. Additionally,
the brain typically gives rise to major cords or nerves that
run to some other part of the body. Not so in echinoderms;
no echinoderm has anything remotely resembling a head or brain.
Those echinoderms that do have a front end or a back end,
and these are mostly sea cucumbers, simply have an opening
for the mouth at one end, and generally the anus opens at
the other end. Although there may be sensory structures around
the mouth that can "taste" the prey, there are no
specific and obvious concentrations of sensory structures
such as eyes, sensory tentacles, or sensory structures that
can detect water-borne chemicals near, or in, this front end.
They just do not have anything remotely resembling a head.
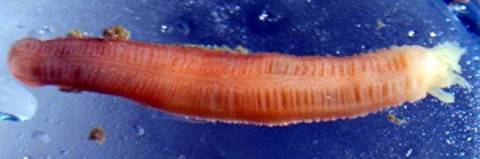 |
Figure 3. Although sea cucumbers, such as this Leptosynapta,
have a mouth at one end
and an anus at the other, they have nothing resembling a head.
Vertebrates, such as fishes, have a brain
that has developed from the front end of a tube of nerves
that lies above the gut. Nerves radiate from the brain and
enervate the regions around the mouth. Sensory structures
are also found in this region. Biologists like to say that
the reason for this type of arrangement is that it is advantageous
for animals to have their sensory structures located in the
front end of the animal. The premise is that it is always
better to see where you are going than where you have been,
to avoid potential problems. Having a sensory array around
the mouth also makes sense; it would seem obvious that organisms
should be able to sense what they are going to eat before
they start eating it. Radially symmetrical animals typically
live as ocean bottom-dwelling animals that are sessile or
relatively slow moving, or pelagic animals. Although they
may be highly predatory, and may even have photoreceptors,
they do not generally hunt their prey visually. Instead, they
may follow scent trails to prey or, like most sea stars, they
may simply move around randomly until they encounter an acceptable
food item.
The arguments about why animals have a
head at the front end are really pretty compelling. Although
almost every mobile animal has such an arrangement, the details
may differ. For example, the vertebrate brain arises from
nerves above the gut, while the brains of most other animals
arise from a nerve ring that surrounds the throat or esophagus.
In these non-vertebrate animals the main nerve cords that
run to the rear of the animal generally pass down the middle
of the bottom of the body rather than as a tube down the back.
Nevertheless, most animals have a head that contains some
sort of light receptors or eyes, some sort of "long-distance"
chemical sniffer, and often, tactile organs such as bristles.
Of course, all of the signals sent by such
sophisticated sensory equipment as eyes and chemoreceptors
need to be decoded and analyzed, which is a primary function
of a brain. Without a brain to figure out what the signals
are, it would be moot to sense them. So, along with the echinoderms'
lack of a brain goes their lack of all of these sensory structures.
Not only do they not have a brain, ANY kind of aggregations
of nerve cells are rare. The lack of evidence of any neural
aggregation that could be a brain, and the absence of any
sort of large sensory structures, could give the impression
that echinoderms blunder through a dark, odorless universe.
Such an impression would be very far from the truth.
Although these animals generally lack large
evident sensory or nervous organs, they are literally covered
in sensory cells of various modalities, often numbering thousands
per square millimeter. While they lack any specific structures
that can be definitely shown to be chemosensory, it may be
said that their entire body is chemosensory. Many echinoderms
have pigmented spots connected to nerves in their body. This
is an arrangement similar to those found in other animals
with true, but primitive, non-image forming eyes. Because
of this structural similarity, the spots found in echinoderms
are referred to as ocelli, or eyespots, but no immediate behavioral
changes can be elicited by applying varying light intensities
to them. Just to make things more interesting, however, many
echinoderms often respond to bright light beams directed anywhere
on their body. It appears that the photoreceptors may record
or signal such things as changes in length of daylight, but
it also appears that the whole body may be able to sense changes
in light intensity, and to trigger behavioral responses to
those changes.
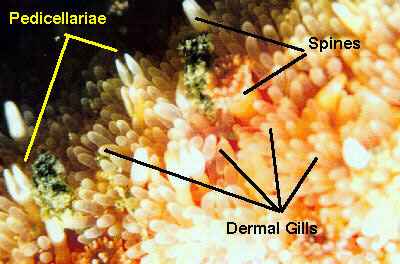 |
Figure 4. A simplified diagram of the plumbing
involved with the basic part of the ambulacral system
of sea star; all other body parts have been removed.
The mouth of the star would be found in the center of
the ring canal. The radial canals would extend out to
the tips of the star's arms.
|
All of this lack of defined nervous structure
not withstanding, many echinoderms have a great deal of relatively
complex behavior. How that behavior is elicited and regulated
without a brain is a question that has been debated for a
number of years among echinodermatologists. Perhaps the best
explanation, given some years ago by Dr. Richard Strathmann,
a noted echinoderm researcher, is that the whole nervous system
may function as an associative and regulatory structure. In
other words, the animal doesn't need a specialized region
to act as a brain, because in a real sense, the whole animal
is a brain.
And It Gets Worse
Or Better
Even
the basic structure of the tissues comprising the echinoderm
body is unusual. Animals typically have connective tissues
and connective tissue proteins that are tough and rugged.
These connective tissues constitute such structures as the
tendons and ligaments of vertebrates and the tough scleroproteins
of invertebrates. When cooked, connective tissues of this
type often have the common name "gristle," a term
that brings to mind a visceral understanding of their consistency
and composition. Well, once again, echinoderms have gone their
own way. Not only do they not have this standard type of connective
tissue, they have, in fact, a unique type of material referred
to as "mutable connective tissue" or "catch
connective tissue." Depending upon the ambient ionic
charge within the fluid spaces of their body, this material
can be rigid and tough, or about the consistency of liquid
gelatin. And it can change from one state to the other in
seconds. (Follow this
link and examine the first image in the article for some
visual evidence of this interesting tissue).
If the mutable connective tissue is in
the "locked" form, such as when a sea urchin spine
is held rigidly upright, it is effectively immobile. When
it is triggered to enter the fluid state in some sea cucumbers,
the animal literally disintegrates as its connective tissue
liquefies. A person holding the animal in his hands at this
time would feel the resultant goo, that a few seconds before
was the sea cucumber, flow through his fingers like thick
mucus.
So far in this column, I have spent some time contrasting
echinoderms with other animals and have generally indicated
that echinoderms don't have this organ or that structure.
There is, however, a limit to the applicability of this sort
of negative comparison. Without something to constitute their
body, they would have to exist simply as a void in space where
nothing else was. As that is patently not the case, just exactly
how are these animals put together, and what unique organs
or structures, if any, do they have? From the point of view
of a biologist who studies comparative morphology, the echinoderms'
whole design is rather peculiar. However, not too much should
be made of that. Depending upon who is doing the counting
and what criteria they are using, there are between 40 and
50 major animal groups called phyla, and each has its own
unique body plan and internal structures. In a sense, the
animals in each such phylum may be said to be "rather
peculiar." Nonetheless, as the reader might begin to
guess by this point, the echinoderm structure is more peculiar
than most.
Where's The Meat?
Animals may be said
to be composed of tissues and spaces, or "voids,"
within those tissues. In some terrestrial organisms, some
of the voids, such as the lungs of vertebrates or the tracheae
of insects, are filled with air, but in most animals, the
voids are filled with fluids. In most marine animals, these
fluid-filled spaces constitute an important secondary component
of each animal's morphology. These spaces have two decidedly
different origins. The largest space in many animals is the
volume inside the gut. In a very real sense, however, the
gut cavity is not within the animal at all. Rather, it is
an elongate section of the exterior environment surrounded
by the gut lining and closed off at either end, rather like
the hole in an elongated donut. The other spaces, the true
body cavities, inside animals are within the actual body structure,
between the external surface of the body's epidermis and the
interior outer surface of the body's gut lining.
In most animals, these body spaces don't define the animal.
They may be relatively large, such as the blood cavity in
arthropods and the body cavity, or "coelom," of
bristle worms, or small, such as the inside of the gonads
of mollusks, but they all share one property: the animal's
shape and the relative "functionality" of the body
are determined by something else, such as the exoskeleton
of arthropods, the shell of most mollusks, or the muscular
body of annelids. Only in a few animal groups, such as the
sipunculans, do the shape and relative composition of the
body cavity exert a primary force in the animal's natural
history.
Of course, by now the reader would probably expect that I
would say that the echinoderms' body cavity exerts a major
influence on all aspects of the animal's biology. I could
say this, but it would be trite to do so. It is really impossible
to single out one organ system or one functional unit of a
living organism and say that this or that structure is more
important than the other. Animals are a functional whole,
and the whole unit and all of its constituent parts need to
be there if the organism is to survive. Nonetheless, the prominence
of the body cavity and its derivitives in the echinoderms,
relative to all other animals, indicates that the elaborations
of these cavities and the resulting structures have been one
of the more interesting themes in their evolution.
Unlike most animals, which have one or two body cavities,
echinoderms have derivitives of at least six. Two of those
six, the body cavity surrounding the gut and the one that
occupies the locomotory organs, are large, and manifestations
of them may be noticeable to reef aquarists who take a close
look at any echinoderms in their tanks. For example, the sea
stars' dermal gills, illustrated in Figure 4 above, are thin
tissue-covered extensions of the body cavity surrounding the
gut, which extend through the body wall and, presumably, act
as respiratory organs. The other cavities are smaller and
although probably no less important to the animal, they are
less apparent.
The bodies of most animals are reasonably firm. They are
composed of tissues and these tissues are durable. In a real
sense, such tissues are the meat and bones of the beast. This
also implies that the body cavities of many animals are pretty
rugged and very stable structures. The abdominal or chest
cavities of mammals are good examples. Although the cavities
may be exposed if they are perforated or cut open, for example
during surgery, the cavities don't collapse. This is because
they are surrounded by layers of strong muscle, connective
tissue, or bone.
Taking these "normal" animals as examples, and
using their types of body cavity to illustrate or conceive
of the cavities in an echinoderm, would be very misleading.
While most animal bodies are comprised of tissues with cavities
within them, echinoderms seem to be built of large cavities
held together and delineated by thin layers of tissue. Such
layers are sufficient to maintain the integrity of the volumes
or systems they surround as long as they are supported by
water. These tissues, however, are thin, filmy and diaphanous,
they are exceptionally easy to break, and if they break or
tear, the animal will often die. Echinoderms are, by and large,
found in marine environments with full strength salinity.
Rapid changes in salinity can result in osmotic imbalances
occurring on either side of these delicate membranes, and
that, in turn, may cause them to rupture. This is why echinoderms
need to be maintained at full oceanic salinity, and it is
also why they need to be acclimated very slowly. Slow acclimation
allows the ionic concentrations on either side of these membranes
to become physiologically balanced. This balancing takes time
but, if done correctly, prevents the membranes separating
the various compartments of the body cavities from rupturing,
and the animal will survive.
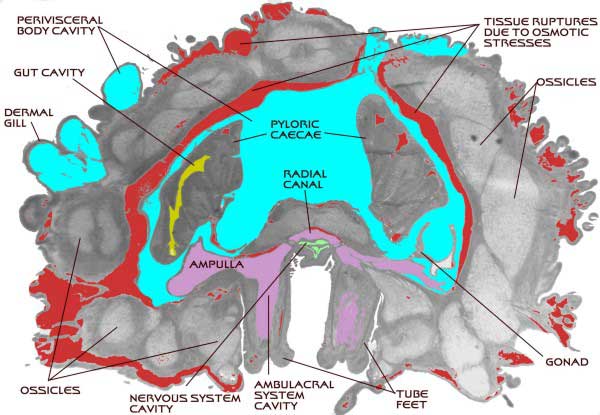 |
Figure 5. This is a modified photomicrograph of a cross-section
of the arm of a small sea star. To make this image, a juvenile
sea star was preserved and then sliced into very thin sections.
The sections were mounted on microscope slides and this one
was photographed through a microscope. Some structures have
been labeled. The ossicles are in the body wall, and the pyloric
cecae are branches off the gut. The ambulacral system is discussed
below. The various parts of the body cavities are colored
blue, purple and green and labeled accordingly. Note the great
extent of the cavity system. This arm was about 6 mm (1/4
in) in diameter. In larger animals the body wall is proportionally
very much thinner and the cavities fill even more of the animal.
Perhaps more importantly, note all the areas in red. These
are places where tissues have been torn during changes in
the salinity that occurred while the animal was being preserved.
Similar changes occur in aquaria if the animals are not acclimated
very slowly, and will result in the death of the animal.
The Hydrovascular System
The body cavity compartment
that occupies the locomotory and food gathering system of
echinoderms is called the hydrovascular, or ambulacral, coelom,
and the system itself is referred to by the two synonymous
terms of hydrovascular or ambulacral systems. This is a complex
system of relatively high-pressure hydraulics that has no
analogue in any other animal. In the barest sense the ambulacral
system consists of fluid-filled tubes and vessels along with
muscular valves to control and isolate portions of itself,
but such a description hardly does it justice.
The ambulacral system is probably best explained in the
context of its anatomy and functionality in a sea star. The
basic system is easy to describe. Inside the animal, and surrounding
the mouth, lies a circular tube called the ring canal, a structure
somewhat like an inner tube. A thin tube, the stone canal,
runs from this canal to the opposite surface of the animal
ending in a perforated calcareous plate called the madreporite,
which is often visible on the upper surface of sea stars.
A long straight tube, called a radial canal, runs from the
ring canal out into each ray. Additionally, large sacs called
"Polian vesicles" also connect to the ring canal;
these may function as hydraulic reservoirs. The radial canals
are blind-ending and terminate at the ends of the rays. All
along the radial canal, pairs of smaller side canals branch
off, one on each side of the radial canal. These small canals
are lined with circular muscles that can contract and close.
Each of these canals terminates in one of the locomotory organs
of a sea star, the tube foot. The tube foot is shaped something
like a pipette or eyedropper. It has a muscular bulb or ampulla
at the top, and a long cylindrical tube projecting out of
the animal. The cylindrical tube has several sets of muscles
surrounding it and terminates in a flat pad lined with adhesive
glands.
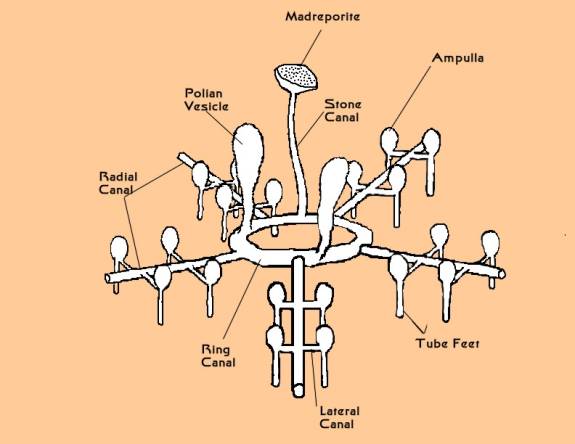 |
Figure 6. A simplified diagram of the
plumbing involved with the basic part of the ambulacral system
of sea star; all other body parts have been removed. The mouth
of the star would be found in the center of the ring canal.
The radial canals would extend out to the tips of the star's
arms.
Locomotion is accomplished by closing down the valve isolating
the tube foot from the radial canal. This isolates the tube
foot as a hydraulic unit. The muscles surrounding the ampulla
relax, and muscles running the length of the tube foot contract.
This shortens the tube foot and pushes the internal fluid
into the bulb at the top of the foot, thereby expanding it.
Small muscles on the side of the foot contract on one side
and relax on the other. This causes the foot to bend in the
direction of the contracted muscle. At this point the muscles
along the length of the foot relax and the protractor muscles
surrounding the bulb contract. This contraction forces fluid
into the tube foot extending it like a small water-filled,
sausage-shaped balloon. As the foot is extending, the small
postural muscles that had been extended along the side of
the foot allowing it to flex, contract; simultaneously, their
previously contracted counterparts relax. This swings the
foot through an arc. At the bottom of the arc, the adhesive
pad contacts the substrate and glues itself to it. As the
muscle causing the swing continues to contract, it pulls the
animal along over the foot. When the foot would leave the
substrate at the beginning of the upswing, other chemicals
are secreted from the adhesive pad and it releases. The foot
now starts to swing upward and the cycle begins anew.
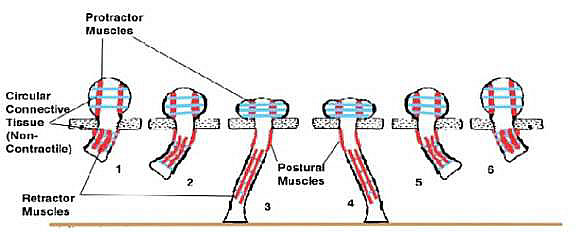 |
Figure 7. The sequence of muscle action
during one cycle of a tube foot. The foot fastens to the substrate
with a glandular secretion in step 3 and releases in step
4.
Think of the coordination necessary to do this with one
tube foot. Then consider that a large sea star may have 40,000
tube feet, all of them working together to move the animal
along. And then consider that this locomotion is all coordinated
and controlled without a brain!
Figure 8. The tube feet on the underside
of the rays of a sunflower star, Pycnopodia helianthoides.
These feet are one external manifestation of the ambulacral
system.
The hydrovascular system is a fluid-filled system, but the
fluid in it is not sea water. The fluid has been actively
pumped into the system through the fine tissues that constitute
the walls of the tubes. This pumping is done by the cells
lining the tissue. They physiologically pump potassium ions
into the tubes and simultaneously pump other ions out. The
pumping results in an internal water pressure that keeps the
tube tightly inflated. Aquarists who forget that sea stars
and other echinoderms need slow, gradual acclimation to salinity
changes often wonder what is wrong with their new pet. They
report that the animal seems "fine," it just doesn't
move. Well, yeah. It can't move. The rapid changes in salinity
have resulted in significant ionic imbalances which rupture
the delicate internal plumbing of the water vascular system.
The animal can't regain the pressure necessary to move, and
it dies in place.
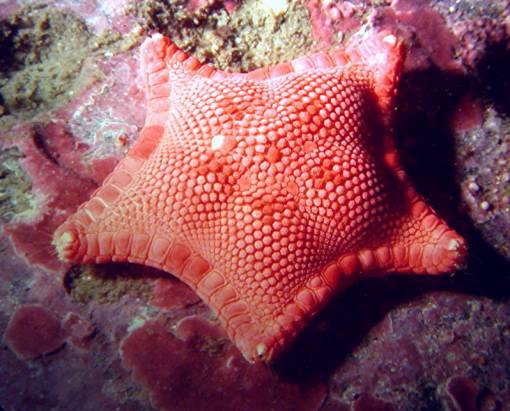 |
Figure 9. A temperate cushion star, Ceramaster arctica.
The white structure near the center of the upper surface is
the madreporite, or sieve plate, which connects the ambulacral
system to the exterior.
Echinoderm locomotion results almost entirely from the ambulacral
system. This is another oddity of the group. Echinoderms are
moderately sized animals, and most animals their size are
highly muscular and move by using some sort of appendages
utilizing lever action. While a few echinoderms, most notably
brittle stars, move almost entirely by direct muscular action,
the vast majority are moved by the muscles in the ambulacral
system.
So, What Makes An Echinoderm?
Echinoderms,
like all animals, are the sums of their parts, and then some.
All of the various oddities of echinoderm structure combine
to create animals that are very odd, and yet, compelling to
the eye. They are just so weird that they are often fascinating.
These are obviously animals like no others.
It might seem that such differences would render them rare
or insignificant. After all, if their evolutionary path led
to important or successful animals, it might reasonably be
expected that there should be a host of copycats, animals
that were "almost sea stars," in the oceans. There
are precedents for just such "copying," which is
termed convergent evolution. For example, the extinct reptiles
called ichthyosaurs, as well as living porpoises and sharks,
all share the same basic body shape. Similarly, hummingbirds,
sphinx moths, and hovering bats all share the same basic shape
and flight pattern, one that facilitates getting nectar and
pollen from deep, trumpet-shaped flowers. Alas, no animals
mimic echinoderms, and there don't seem to be any close relatives
of the group as a whole. Though distantly related to animals
such as vertebrates on one extreme and corals on the other,
they are really unlike any other group. Additionally, there
appear to be no examples of convergent evolution toward an
echinoderm form by any other group.
This might indicate that they are rare
and unimportant. In fact, the situation is just the reverse.
They are often very abundant and in most marine sea bottom
communities, they are THE dominant animals. In many
ecosystems, their activities structure and maintain all other
animal populations. Furthermore, they have maintained this
level of ecological dominance for a very long time, indeed.
Echinoderms have been the dominant life forms on the ocean
bottoms for at least 300,000,000 years, and there is no sign
that that is about to change anytime soon (Tasch, 1973).
Figure 10. The grazing of Diadema sea urchins
has been shown to be extremely important in the structuring
of coral reefs. If the urchins are removed from a reef, the
reef may change from a coral dominated area to one dominated
by algae in a very short time period. (See: Knowlton, 2001).
|
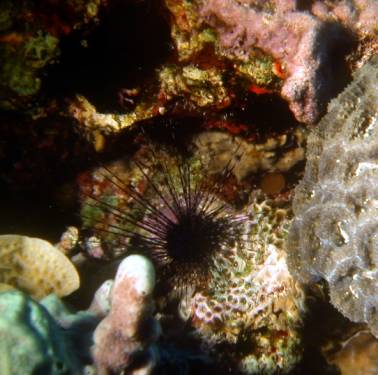 |
Next month, I will discuss the diversity
of echinoderms with some brief notes on how to maintain some
of the common forms in aquaria. In nature, the coral reefs
we attempt to emulate in our aquaria are largely maintained
in the form we are familiar with by the actions of many echinoderms,
and they have a place in many aquaria as well.
|