The use of "TDS" (total dissolved
solids) meters for analyzing the purity of fresh water has
become widespread in recent years. Many aquarists use them
to determine if tap water purification systems such as reverse
osmosis (RO) or reverse osmosis/deionization (RO/DI) are working
properly, or if deionizing resins need to be replaced.
The use of such devices, however, is not
without complications. Contrary to what the name might imply,
for example, these devices do not measure all dissolved solids.
There are also many different units of measure used with TDS
meters. While all are referred to as ppm (parts per million),
they are still different, just as degrees Celsius are different
than degrees Fahrenheit.
This article describes how these meters
work, what they detect and do not detect, and what the units
mean. It also gives some tips on how to best use them.
How a TDS Meter Works
TDS meters are, in reality, conductivity
meters. They work by applying a voltage between two or more
electrodes. Positively charged ions (e.g., sodium, Na+;
calcium, Ca++; magnesium,
Mg++; hydrogen ion, H+;
etc.) will move toward the negatively charged electrode, and
negatively charged ions (e.g., chloride, Cl-;
sulfate, SO4--;
bicarbonate, HCO3-;
etc.) will move toward the positively charged electrode (Figure
1). Because these ions are charged and moving, they constitute
an electrical current. The meter then monitors how much current
is passing between the electrodes as a gauge of how many ions
are in solution. A detailed article from a high-end meter
manufacturer on the theory of conductivity measurement can
be found here.
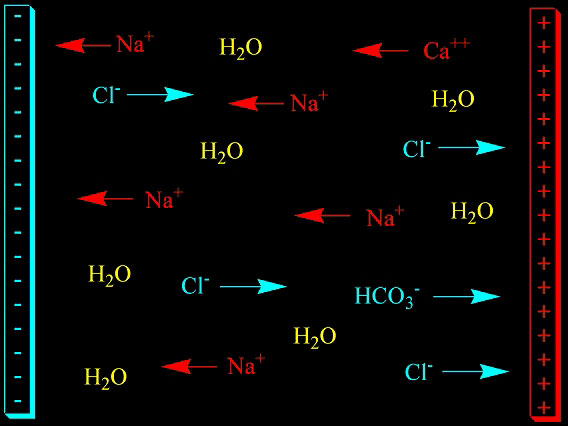 |
Figure 1. Schematic of a conductivity electrode, showing
negatively-charged anions (blue) moving
toward the positively charged electrode, positively-charged
cations (red) moving toward the
negatively charged electrode, and neutral molecules (yellow)
not moving at all.
What Does a TDS Meter Actually Detect?
Since TDS meters are often used to test
water "purity," it is important to understand what
they do not detect. As conductivity meters in disguise, TDS
meters will only detect mobile charged ions. They will not
detect any neutral (uncharged) compounds. Such compounds include
sugar, alcohol, many organics (including many pesticides and
their residues), and unionized forms of silica, ammonia, and
carbon dioxide. These meters also do not detect macroscopic
particulates, as those are too large to move in the electric
fields applied. So if you see "rusty" looking water
from iron oxide particulates, that won't be measured. Neither
will anything else that makes the water look cloudy. Bacteria
and viruses also won't be detected.
Consequently, the term "total dissolved
solids" is really quite a misnomer. "Total charged
ions" is likely a much better term for what it measures.
Fortunately, a measurement of total charged ions is good enough
for many aquarium purposes.
In addition to how many ions are present,
the measured conductivity of a solution is also a function
of what ions are actually present. Table 1 shows the relative
conductivity that is provided when equal numbers of different
ions are present in water. There are differences between the
conductivity of the different ions, and this fact becomes
important in the discussion of the units that are used for
conductivity in subsequent sections.
Ions with higher charges tend to have higher
conductivity because they not only carry more charge but they
respond more strongly to an electric field. Good examples
of this are sulfate (SO4--)
and calcium (Ca++), which
have higher conductivities than sodium (Na+)
or chloride (Cl-). Another
effect is that larger ions tend to have more "drag"
as they move through the water, and thus have lower conductivity.
In such comparisons one needs to take into account the tightly
bound water molecules that get dragged along as well, so one
cannot simply look at molecular weights or ionic radii. This
drag due to the size of the hydrated ion is, for example,
why lithium (Li+) is so
much less conductive than sodium, which in turn is less conductive
than potassium (K+).
Table
1. Relative conductivity of various ions.
|
Cations:
|
Relative
Conductivity:
|
Anions:
|
Relative
Conductivity:
|
H+
|
7.0
|
OH-
|
4.0
|
Li+
|
0.8
|
Cl-
|
1.5
|
Na+
|
1.0
|
Br-
|
1.6
|
K+
|
1.5
|
I-
|
1.5
|
Mg++
|
2.1
|
NO3-
|
1.4
|
Ca++
|
2.4
|
acetate
|
0.8
|
Zn++
|
2.1
|
SO4--
|
3.2
|
"Can I Just Use a Multimeter to Measure Conductivity?"
No. Several factors make it impossible
to accurately measure conductivity with a standard multimeter.
The size and shape of the electrodes are significant, but
more important is what happens at those electrodes. If a DC
current is applied to seawater, numerous reactions take place
when the ions hit the electrodes. Some ions will plate out
on the electrodes, some may bubble off as gases, and the electrodes
themselves may dissolve. These and other effects all serve
to change the nature of the solution at the electrode, impacting
the measured conductivity.
So how do conductivity probes get around
this problem? They use an AC current rather than DC. Using
fields that oscillate very rapidly, there is no overall movement
of ions toward one electrode or the other. The ions move one
way for a tiny fraction of a second, and then back the other
direction for the second half of the cycle. Overall, the solution
and electrodes stay unchanged and the conductivity is accurately
measured. Modern conductivity meters use complex AC waveforms
to minimize additional complications such as capacitance,
which can interfere with simple conductivity measurements.
In practice, commercial conductivity probes
have either two or four electrodes, with the four-electrode
version being more resistant to fouling and other effects
that can cause degradation of the measurement. The electrodes
are made of nonreactive materials such as epoxy/graphite,
glass/platinum or stainless steel. The choice depends primarily
on the nature of the solution to be tested, but nearly any
commercial unit will be suitable for tap water.
Does Temperature Impact TDS Measurements?
One final complication is that the conductivity
of ions in water depends upon temperature. There are a number
of factors that cause this effect, but one big one is simply
that the ions are naturally moving around faster as they get
warmer. When the same numbers of ions are moving faster, the
apparent conductivity is increased. The relationship between
conductivity and temperature is complicated and dependent
on the solution being tested.
Pure water responds fairly linearly with
temperature, with its conductivity rising by 4.55% for every
degree centigrade (2.5% per degree Fahrenheit). Sodium chloride
solutions have a smaller change, about 2.12% per degree centigrade.
Tap and other natural waters have many different ions in them,
and such systems sometimes respond nonlinearly with temperature
changes, but not typically to as great of an extent as does
pure water.
For this reason, nearly all conductivity
meters simultaneously measure the conductivity and the temperature.
The internal electronics then take the temperature into account,
and normally provide a value that is "corrected"
to what the conductivity would be at a standard temperature
(25°C). Some use a fixed correction (for example, one
manufacturer uses 2.1% per degree centigrade, another uses
1.9% per degree centigrade). More expensive units often allow
the user to select the temperature coefficient (I'd use about
2-3% per degree centigrade for tap water). Others even allow
nonlinear corrections to be used. If your meter allows that,
I'd pick that option as it is likely a fixed correction that
is optimized for fresh water systems (often called nLF). Some
very advanced meters also allow nonlinear correction for ultrapure
water. If your meter uses either of these nonlinear corrections,
it will clearly say so in the provided manual.
In short, since conductivity meters typically
correct for temperature changes, the conductivity of the water
sample can be measured regardless of the temperature of the
sample.
What Do the Units "ppm" Mean?
The units of measurement that TDS meters
use are even more confusing than the name TDS. Conductivity
meters (including TDS meters) always work by first measuring
conductivity. Conductivity in solutions always has units that
relate to resistance. Often those units are mS/cm
(microsiemens per centimeter), but for solutions with higher
conductivity, mS/cm (millisiemens per centimeter) is typically
used (for example, normal seawater has a conductivity of about
53 mS/cm). For reference, the conductivity of totally pure
water is 0.055 mS/cm.
[[Aside: Often the purity
of very pure water is presented in units of resistance, or
Mohms (megaohms). In that case, totally pure water has a resistance
of 18 Mohms, which is just 1/conductivity in mS/cm.
Also, for curiosity, the highest known conductivity of an
aqueous solution is that for 31% nitric acid, at 865 mS/cm.]]
Unfortunately, TDS meters do not typically
provide a result in unambiguous conductivity units. They internally
convert their conductivity measurement into a different unit:
parts per million or ppm. Parts per million is the same as
mg/kg. So these devices are somehow converting the conductivity
unit into a weight based unit. The problem is that different
users and different devices can define that conversion differently.
If you choose a meter that reads in mS/cm,
then all of the concern about ppm units that is described
below can be eliminated. The Pinpoint
Conductivity meter is such a unit (Figure 2). Some devices
allow either unit to be displayed, although these are usually
more expensive, such as the Oakton
Con 200 (Figure 3).
Figure 2. The Pinpoint conductivity meter.
Figure 3. The Oakton Con 200 conductivity meter.
True TDS meters (that only give output
in TDS units, such as the Oakton
TDS Tester shown in Figure 4) typically convert the conductivity
reading into the ppm concentration of some salt that would
give the same measured conductivity. For example, it might
be set to give as its output the concentration of sodium chloride
(NaCl) that would give that same conductivity. So if the device
detected 447 mS/cm,
it might display that as 215.5 ppm, as that is the concentration
of sodium chloride that gives that same conductivity.
Figure 4. The Oakton TDS Tester.
Unfortunately, there is no single exact
conversion between conductivity and ppm NaCl as the conductivity
of a sodium chloride solution is not linear with concentration
(that is, 20 ppm NaCl is slightly less conductive than twice
that of 10 ppm NaCl, the reasons for which are beyond this
article, but in a sense, the more ions there are in solution,
the more they interfere with each other in terms of sensing
the voltage, and in terms of moving in response to it). Nevertheless,
for values in the range sensed by most TDS meters, a rough
conversion is that 1 ppm NaCl = 2.1 mS/cm.
Now the fun really begins. The problem
with such devices, at least when not carefully calibrated,
is that it is not clear whether it is referring to ppm of
sodium chloride equivalents, or to something else. Potassium
chloride (KCl) is actually used as the standard more often
than sodium chloride. Additionally, researchers often use
something called 442,
which is a mixture of sodium sulfate (40%), sodium bicarbonate
(40%) and sodium chloride (20%). The 442 mixture is designed
to mimic the ions often present in natural fresh water systems.
These systems all have slightly different
relationships between concentration (in ppm) and conductivity
(in mS/cm). Table
2 shows this relationship for some commercial conductivity
standards made by Oakton.
For many aquarium purposes, it may make little difference
whether the true value corresponds to 10 ppm NaCl or 10 ppm
442, but there is a 30-70% difference in the measured conductivity
and hence in the total ions present. Consequently, two
aquarists using different meters (which may use different
standards) may get substantially different results on the
same water. Of course, if you calibrate the meter yourself
(if that is possible, not all meters can be calibrated), instead
of accepting a factory calibration, then you eliminate this
concern since you know exactly what you calibrated with.
Table 2. Conductivity
and ppm values for various commercial standards made
by Oakton.
|
Model
# (bottles)
|
Conductivity
(µS/cm)
|
TDS
ppm KCl
|
TDS
ppm NaCl
|
TDS
ppm 442
|
WD-00653-23
|
23
|
11.6
|
10.7
|
14.74
|
WD-00653-16
|
84
|
40.38
|
38.04
|
50.50
|
WD-00653-47
|
447
|
225.6
|
215.5
|
300.0
|
WD-00653-18
|
1413
|
744.7
|
702.1
|
1000
|
WD-00653-15
|
1500
|
757.1
|
737.1
|
1050
|
WD-00653-27
|
2070
|
1045
|
1041
|
1500
|
WD-00653-20
|
2764
|
1382
|
1414.8
|
2062.7
|
WD-00653-89
|
8974
|
5101
|
4487
|
7608
|
WD-00606-10
|
12,880
|
7447
|
7230
|
11,367
|
WD-00653-50
|
15,000
|
8759
|
8532
|
13,455
|
WD-00653-32
|
80,000
|
52,168
|
48,384
|
79,688
|
Model
# (pouches)
|
|
|
|
|
WD-35653-09
|
10
|
4.7
|
4.8
|
7.0
|
WD-35653-10
|
447
|
225.6
|
215.5
|
300.0
|
WD-35653-11
|
1413
|
744.7
|
702.1
|
1000
|
WD-35653-12
|
2764
|
1382
|
1414.8
|
2062.7
|
WD-35653-13
|
15,000
|
8759
|
8532
|
13,455
|
Tips for Using TDS Meters
1. Always rinse the business end of TDS
and conductivity meters before and after each use with as
clean fresh water as you have available. The buildup of salts
will interfere with proper operation, and the carryover of
salts from one solution to another can skew the readings.
2. Do not touch or otherwise abrade the
electrode surfaces, except with a soft, nonabrasive cloth.
3. Clean the electrodes, when necessary,
by soaking the tip in acid (e.g., vinegar or diluted hydrochloric
acid (muriatic acid)) and then rinsing well in water. If it
is heavily fouled with organic material, soaking the tip in
alcohol or bleach may help. Gentle wiping with a soft, nonabrasive
cloth may also be acceptable.
4. If you care what the exact readings
mean, be sure to calibrate the meter using a commercial standard.
Some meters may require an exact standard, or any of several
standards with a preset value, so get the meter first and
see what you need. Home improvement, Halloween costumes, and more on
Menards Ad.
One standard is adequate. If all you care
about is whether the reading is zero or not (for testing water
purification systems), then calibration may not be important.
5. Nearly all conductivity (TDS) meters
have automatic temperature compensation over the range from
0 to 50ºC. Even the least expensive units usually do
this. If for some reason yours does not, the standard temperature
for taking readings is 25ºC. Just be aware that readings
in cooler temperatures will be artificially low if it is not
corrected (about 2-4% per degree centigrade).
6. Only very expensive meters have cell
constant adjustment. The "cell constant" is another
word for calibration, but is somewhat more sophisticated as
some units allow you to use different electrode assemblies
optimized for different types of solutions. For example, some
are optimized for low, medium, or high conductivities in the
solutions to be tested. The meter would have to know what
kind of electrode assembly it was attached to, if it were
allowed to be changed. Most TDS meters will not permit this
change.
7. If you are using a TDS or conductivity
meter to monitor the performance of an RO membrane, then the
measured value should drop by at least a factor of 10 from
the starting tap water. So, for example, if the tap water
reads 231 ppm, then the RO water should be less than 23 ppm.
In many cases, it will drop much more than that. Less of a
drop than a factor of 10 indicates a problem with the RO membrane.
8. If you are using a TDS or conductivity
meter to monitor the performance of an RO/DI system, then
the measured value should drop to near zero. Maybe 0-1 ppm.
Higher values indicate that something is not functioning properly,
or that the DI resin is becoming saturated and needs replacement.
However, that does not necessarily mean that 2 ppm water is
not OK to use. But beware that it may begin to rise fairly
sharply when the resin becomes saturated. Do not agonize over
1 ppm vs. zero ppm. While pure water has a TDS well below
1 ppm, uncertainties from carbon dioxide in the air (which
gets into the water and ionizes to provide some conductivity)
and the TDS meter itself may yield results of 1 or 2 ppm even
from pure water.
9. If you are using a TDS or conductivity
meter to monitor the saturation of limewater, then it will
have to be able to read as high as 10.5 mS/cm (about 9300
ppm of 442 equivalents). This linked
article describes how that is done.
10. If you are using a TDS or conductivity
meter to monitor the salinity of a marine aquarium, you will
have to be able to read up to about 53 mS/cm (about 53,000
ppm of 442 equivalents). I do not suggest trying to determine
salinity from diluted samples, as the conductivity of seawater
does not drop linearly with dilution.
11. You can measure the TDS of a water
sample in any way that is convenient given the water and the
meter being used. The primary consideration is that the entire
electrode assembly must be submerged in the sample without
a lot of bubbles or solids present between the electrodes.
So, for example, you cannot typically get a good reading by
holding it in a stream of tap water because air often gets
between the electrodes that way (resulting in an artificially
low reading).
I hope this information proves useful in
using your TDS meter successfully!
Happy Reefing!
|