Even though they may be caring for relatively
large numbers of invertebrate animals, most aquarists have
significant misconceptions about these creatures. Invertebrates
are often seen as a single group of animals, similar in many
regards to the more familiar vertebrates such as dogs, cats
or fishes; such a conception is both wrong and misleading
and can result in significant problems in caring for them.
Even the basic separation of animals into invertebrates and
vertebrates is misleading as it implies some sort of division
into equal parts. The total number of animal species is not
known, but estimates of the number range from about 2,000,000
species to about 50,000,000 species. The number of vertebrate
species is relatively well known and stands at about 45,000,
thus that group comprises between 0.09% to about 2.25% of
the total number of living animal species. In terms of species
numbers, vertebrates are rather insignificant.
The inclusion of humans within vertebrates
probably drives the separation of the animal kingdom with
an "us" and "them" division, with most
people assuming that the invertebrates are just "not
as good" as the vertebrates. In truth, the vertebrate
body plan IS a rather good one, and probably the best design
for making large animals. However, most animals are neither
large nor vertebrates, and most have a basic architecture
that is fundamentally and distinctly different from that found
in the vertebrates. The inclusion of humans within the vertebrates
has one other, more important, consequence: most people tend
to think that invertebrates are just simpler, smaller versions
of vertebrates. In truth, they are fundamentally different
types of life. I thought I would use this column to explore
a few of these differences.
See The Differences
Humans are possibly the most visually oriented
of animals. We have a visual system that is second to none;
our eyes have exceptional powers of resolution and we can
see colors. Outside of the other large primates, no other
animals possess eyes that are as capable. Some of the raptors
can resolve finer objects, but they live in a monochrome world,
and lose all the visual information that is encoded in color.
Because of our reliance on vision, we tend to think that all
other animals, even those with good eyes, are equally dependant
upon vision. Such just isn't the case; for most animals, vision,
as we perceive it, is simply irrelevant. Most animals sense
their worlds very differently than we do ours.
Figure
1. All of the bristles on this female caprellid amphipod's
body are sensory. They respond to
water moving over them, and they are hollow and contain nerves
that chemically sense the water
for critical dissolved materials.
We often discuss water flow and how it
influences the animals in our systems. Well, folks, as hobbyists
we really don't have a clue how it influences them. These
animals are living in a system of moving fluids that interact
with sensory receptors all over their body surfaces. Most
of the animals in aquaria come from environments where the
water moves in non-turbulent bulk, a more-or-less laminar
flow. Such animals have been shaped by millions of years of
natural selection to be able to exist in such an environment.
These environments have specific properties of water movement
and, for optimal health, the organisms require water movements
to conform to the conditions in their natural habitat. Unfortunately,
we cannot even approximate such bulk flow in any aquarium.
The results of this failure on our part are the abnormal growth
forms and behaviors seen in many sessile marine invertebrates
in our systems. Most sponges in our systems grow abnormally
- if they grow at all. Corals and soft corals are also typically
misshapen. Such animals often cannot feed or eliminate wastes
normally, and they may be subject to abnormal microhabitats
with all of their associated problems, such as unusual chemical
distributions, lighting patterns, and feeding restrictions,
simply because the water flows in a turbulent rather than
a laminar manner.
And then there are eyes
. When most
aquarists look at an invertebrate with what appears to be
eyes, they see something that is not there. They generally
see an animal that they perceive can see them as well as they
can see it. With very few exceptions, such a perception is
totally false. There are some invertebrates that have camera
eyes that have the ability to resolve small difference as
well as a vertebrate eye of the same size. Such eyes are found
in the cephalopods, such as squids and octopuses, a few snails,
and a few polychaete worms. No other eye in the marine invertebrates
is capable of forming what we would call a recognizable image.
Probably the most common type of "eye"
found in the marine invertebrates is a simple ocellus or "eyespot."
This photoreceptor consists of a group of pigment cells and
group of sensory neurons. The pigment cells are often found
in the shape of a cup or bowl, and the sensory receptors are
often located within the bowl. Such a photoreceptor acts to
shade the sensory cells from light coming from most or some
directions. Because of this, these receptors are often considered
to be directional receptors or a sensory receptor that allows
the animal to move toward or away from light.
Figure
2. Left is a Polyclad flatworm. The brain is visible
as a pair of large white patches to the left
side of the animal. Small eyespots are visible upon them.
Right is a close up of the eyespots. These
are simple ocelli and probably allow the animal to sense light
and its direction.
Interestingly enough, however, varying
the light on many of these photoreceptors seems to have no
discernable effect whatsoever. This probably means we don't
know what to look for with a specific response, but it could
also mean that the photoreceptor acts as a light "accumulator."
Such an organ might, for example, produce a small amount of
a hormone while it is illuminated. If such a hormone was destroyed
at a constant rate by the body's metabolism, the hormone would
gradually accumulate with increasing day length in the spring
and gradually diminish during the autumn, and hormone levels
could be used to synchronize spawning or other behaviors.
The most successful and widespread photoreceptor
found in the invertebrates is the compound eye. These are
eyes made of several to several thousand discrete functional
units called ommatidia. Each of these ommatidia, in turn,
is made of several discrete cells and function as independent
photoreceptor units. They are not focusable, and do not form
images on a retina. Instead, the image is focused on a central
sensory structure running the length of the ommatidium. Consequently,
any item impinging on the photoreceptor's field of view, regardless
of its distance from the lens, triggers a response. Detection
of distances by such an eye is impossible, but such an eye
is extremely sensitive to objects moving across the field
of view. The visual field of each unit of a compound eye is
typically small; the whole eye's aggregated image would be
pixilated and analogous to a small cathode ray monitor with
very large pixels. While incapable of forming a fine image,
such a photoreceptor would be very well adapted to detecting
movement by the continual flickering of ommatidia on and off
as objects passed through their small discrete units.
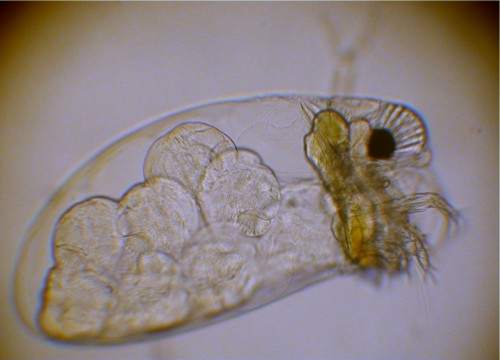 |
Figure
3. A marine water flea, Evadne. The units of the
compound eye are visible to
the right. The black area is the region of the photoreceptor
chemicals, but the tubular
nature of the compound eye units or ommatidia extends upward
from this region.
Many of these animals with compound eyes
can see colors. In some cases they can even see the same ones
we do. On the other hand, some animals have a decidedly different
way of looking at the world. Color vision is the result of
the brain integrating the responses of visual pigments, called
rhodopsins, each responding to distinctly different wavelengths
of light. Most animals with color vision have three visual
pigments generally responding to colors near magenta, yellow
and cyan. The processing of the responses from these photoreceptors
produces what we (and most other animals with color vision)
perceive of as our colorful world. Well, not in the much maligned
mantis shrimps. Some of these superb predators have been reported
to have eyes containing as many as 16 different types of photoreceptors,
color filters and polarization receptors. They possess 12
narrowly tuned spectral sensitivities that cover the color
spectrum. The human eye can respond easily to around 30,000
different colors and hues. Mantis shrimps could conceivably
respond to millions of discrete colors, most of which would
be impossible for us to even distinguish.
For some more information and illustrations
of a mantis shrimp eye click here.
Even the animals with good camera eyes,
such as the octopuses and squids, don't see things the way
we do. Vertebrate eyes are indirect eyes, the photoreceptor
cells, the rods and the cones, face away from the incoming
light and in effect respond to the light bouncing off a reflective
layer at the back of the retina called the tapetum. This reflective
layer is differently colored in various vertebrates and is
what is responsible for the various colors that vertebrate
eyes reflect in the dark. Be that as it may, the camera eye
of cephalopods and vertebrates produce images in much the
same manner. However, the two groups of animals do not perceive
the same image in the same manner. Much of the processing
of an image is done by neural units, sort of like subprocessors,
that integrate the responses from the photoreceptor cells,
prior to the responses being sent to the brain.
The neural subprocessors, or retinal ganglion
cells, of the cephalopod and vertebrate eyes are connected
to their receptor cells in decidedly different manners. This
results in an image of the same object being perceived of
differently. What a vertebrate might perceive as a solid black
circle, for example, might be seen by an octopus as a series
of checkerboard patterns of alternating light and dark oblique
lines. So, even these two eyes might seem similar, they are
sending fundamentally different signals to the respective
brains.
"Prick us, do we not bleed
.blue?"
Everyone is familiar with the circulatory
system of vertebrates consisting of a heart which pumps nice
red blood through arteries to the tissues and thence through
capillaries which carry blood through the tissues and finally
into the veins to bring blood back to the heart. This circulatory
system is basic to the vertebrates and to the polychaete or
bristle worms which have essentially the same pattern. The
worm system is an independent variation on the theme, however,
as they use a different type of hemoglobin and it is not in
corpuscles but free in the blood.
The circulatory system in most invertebrates
is wholly unlike that seen in vertebrates. Circulatory systems
exist to distribute dissolved materials throughout an animal's
body. Specifically, they distribute dissolved foodstuffs such
as sugars and amino acids from the guts to the tissues where
they are used, and they move dissolved gases to and from points
of utilization in the tissues to gas exchange surfaces such
as gills, and they move nitrogenous waste products to the
excretory organs. That one system can perform all of these
tasks simultaneously is marvelous, but equally marvelous is
the diversity of different designs that do it successfully.
If the circulatory system is looked at
conceptually, there are really two extremes. One extreme is
the pattern seen in the vertebrates and polychaete worms.
Here, the blood is contained totally with in vessels. The
other extreme is seen in animals such as peanut worms or sipunculans.
These animals really lack a dedicated circulatory system,
but the entire body is a blood filled bag, and the contents
just "slosh" around as the animal moves.
Somewhat in between these extremes is the
pattern seen in the crustaceans. These animals have a heart
that generally is found in the upper central portion of the
back. This heart pumps blood forward to the animal's brain
through thin walled vessels. From then on the blood flows
through channels or spaces in the tissues. Although the blood
is not constrained by vessels, the blood flow is not sloppy
or haphazard, but is rapid and precise. Crustaceans typically
have more blood per mass of the animal than do vertebrates,
but for animals of a comparable size the velocity of blood
within the tissues is about the same in both groups.
Crustacean blood differs from vertebrate
blood in one other characteristic. It is not red as it lacks
hemoglobin. Hemoglobin in vertebrate blood carries dissolved
oxygen to the tissues and carbon dioxide to the respiratory
surfaces. In most small crustaceans, these gasses are simply
dissolved in the blood and the relatively larger volume of
blood ensures that sufficient gas exchange occurs. In larger
crustaceans, a chemical called hemocyanin is found dissolved
in the blood. Hemocyanin is a material made of protein subunits
complexed with copper ions. It will bind to oxygen in areas
of high oxygen concentration and release it in areas of lower
oxygen concentration, so it is a respiratory pigment. It is
not a particularly good respiratory pigment when compared
to hemoglobin, but it does appear to assist in oxygen transport.
Like hemoglobin, its color changes depending upon whether
or not it is carrying oxygen. Lacking oxygen it is colorless,
but while carrying oxygen, it is a beautiful pale blue or
cyan; which, of course, gives the pigment its name.
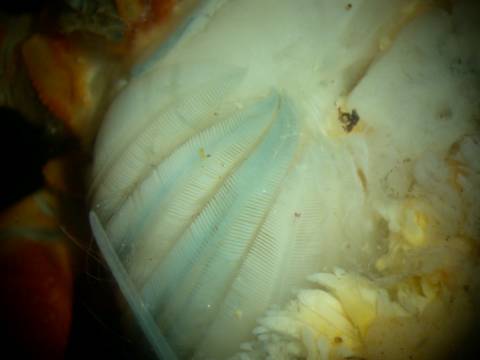 |
Figure
4. The gills of a Northeastern Pacific shore crab, Hemigrapsus
nudus, exposed in
a dissection. The blue color in the gills is due to the hemocyanin
in the crab's blood.
The blue blood of the horseshoe crab Limulus
polyphemus is extracted and used in biomedical research.
For a picture of such blood, follow this link.
Hemocyanin is also found as a respiratory
pigment in mollusks, and in cephalopods there is a blending
of the closed circulatory pattern such as is found in vertebrates
with the blood pigment of the crustaceans and other mollusks.
The design of the circulatory and respiratory systems of the
cephalopod mollusks such as squids and octopuses is the most
efficient design found in any water-breathing animal. Fishes
have a closed circulatory system, but it is a low pressure,
relatively slowly flowing system. The fishes pump their blood
through the gills immediately after leaving the heart. This
blood flows through a capillary bed in the gills and then
has to flow through the body, but blood pressure is lost in
the capillary bed of the gills and from there back to the
heart the flow is slow. It wasn't until the double pump heart
of the advanced reptiles, birds, and mammals evolved that
a high pressure rapid circulatory system evolved in the vertebrates.
Such a system isolates the respiratory capillary bed, in the
lungs in this case, from the rest of the general body circulation.
The general body, or systemic, circulation is high speed and
high pressure allowing for the development of high-pressure
filtration kidneys and rapid transport of nutrients and gases,
which in turn allowed the development of the high metabolic
rate characteristic of mammals and birds.
In the seas, a similar circulation pattern
is seen in the cephalopods, only it involves three hearts.
Each of the two gills has a heart at its base pumping blood
through the capillary bed in each gill. From the gill the
oxygenated blood flows to a third, systemic, heart which pumps
blood to the brain and body. As with the mammals and birds
on land, this high pressure system has allowed the development
of a higher metabolic rate and efficient high-pressure kidneys.
Probably as a result of this efficiency, the evolution of
a large nervous system with obvious intelligence has been
favored. The intelligence of the cephalopods is well known;
but it is from a different blue-blooded base than our own.
Interestingly as we learn more about it some functional convergent
similarities have been found. For example, using criteria
developed for primates, octopuses have recently been shown
to play with toys (Mather & Anderson, 1999). Perhaps at
the basic level of information processing, it doesn't matter
what color your blood is or the source of your neuronal information,
once a basic threshold is crossed some properties of intelligent
animals may have to share certain functional attributes such
as the need, occasionally, to play.
|